Compounds
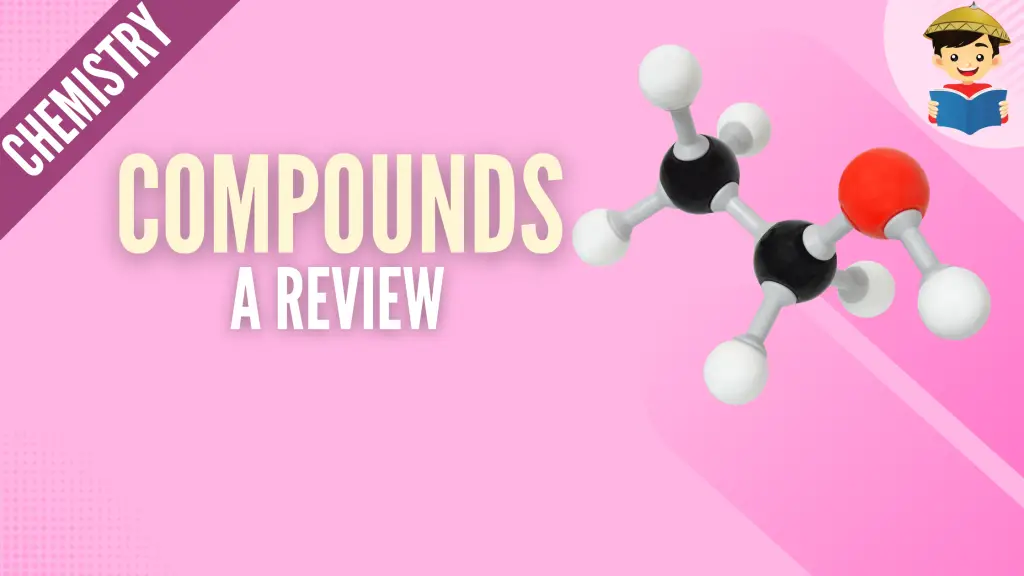
Have you heard of any advertisement claiming their product is “chemical-free?” How did you react?
Well, for chemists, that is an example of a very bad and misleading advertisement. Why? It’s because there’s no such thing as “chemical-free.” A chemical is a substance that can be represented using a fixed chemical formula and possess characteristic properties.
A compound is a type of chemical which is defined as a substance composed of atoms of two or more elements chemically united in fixed proportions. The water we drink and even the air we breathe are all considered “chemicals.” Learn more about “chemicals” and compounds in this section!
Click below to go to the main reviewers:
Table of Contents
- Types of Chemical Bonds
- How To Name Compounds and Write Compound Formulas
- Determining Oxidation Number
- Representation of Compounds
- Writing Lewis Structure and Molecular Geometry
- Intermolecular Forces of Attraction
- References
- Download Article in PDF Format
- Test Yourself!
Types of Chemical Bonds
1. Metallic Bond
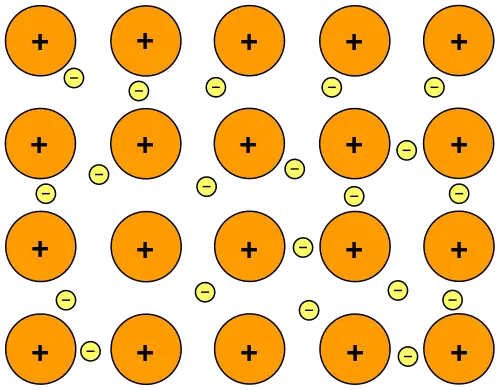
This is a type of chemical bond that forms among metallic substances and is a direct consequence of attractive electrostatic forces between the negatively charged sea of delocalized electrons and the positively charged metal ions.
In solid metals, individual metal atoms are so close to one another that the electron cloud of one metal atom overlaps with its neighboring metal atom. This creates the “sea of delocalized electrons.” In other words, the electrons are free to wander within the crystal structure.
2. Covalent Bond
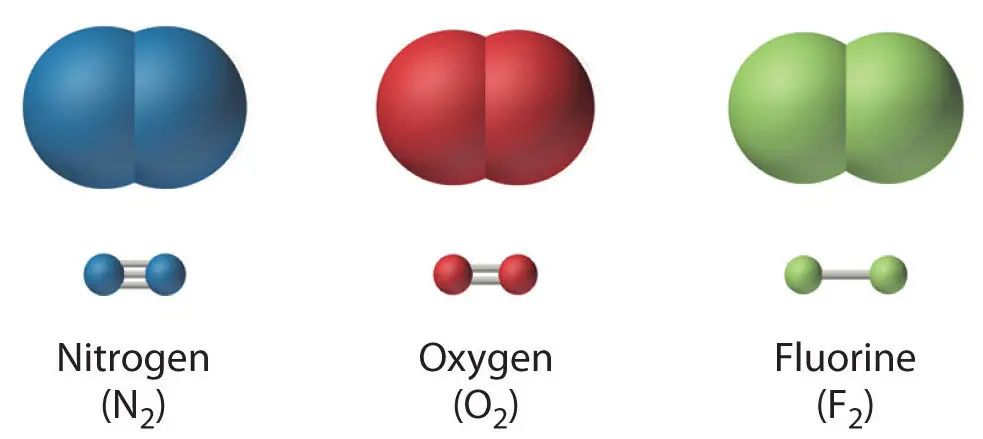
A covalent bond is a chemical bond wherein the electrons involved in bond formation are shared by the two atoms bonded with one another. As you know, a covalent bond is designated by a “dash” connecting one atom to another. Each covalent bond is composed of two electrons, and each atom being bonded covalently contributes one electron to that bond.
A covalent bond can be a single (ex: F2), double (ex: O2), or even triple (ex: N2) bond.
3. Ionic Bond
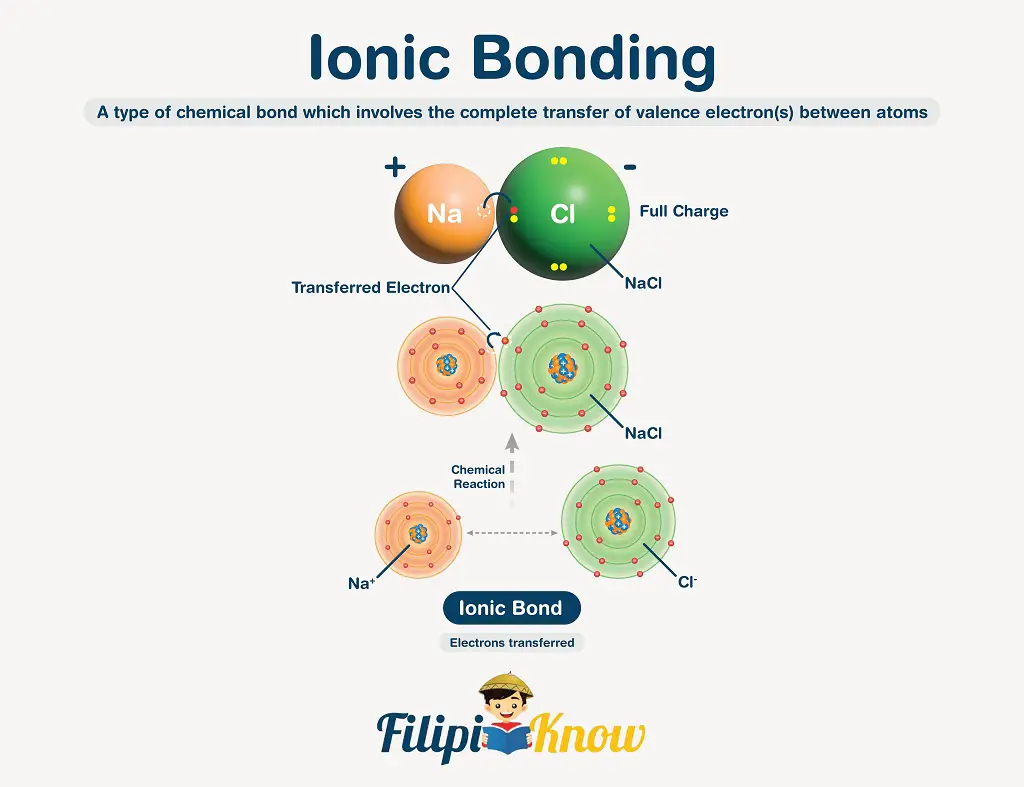
An ionic bond is a chemical bond involving the complete transfer of valence electron(s) between atoms. This bond usually occurs between a metal and a nonmetal.
The transfer of electrons is made possible because metals tend to lose their valence electron(s) to become a positively-charged cation. In contrast, the nonmetals tend to gain the electron(s) lost by the cations to become negatively-charged anions.
In writing ionic compounds, both ions are enclosed inside a square bracket, with their charge and numerical coefficient (if there is any) written outside the bracket. See the figure below.
4. Coordinate Covalent Bond
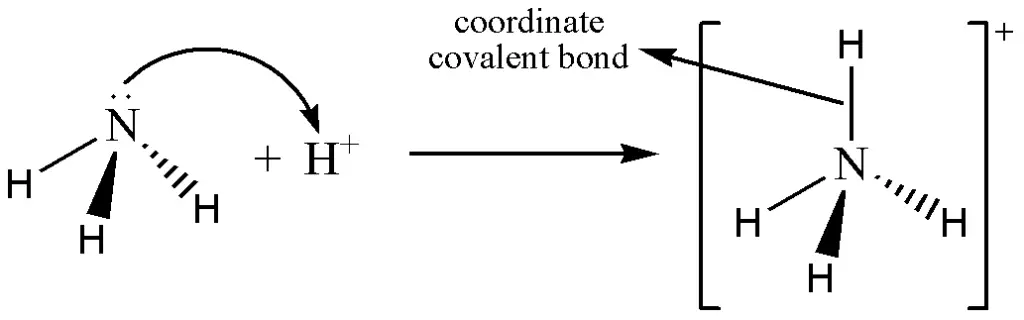
The coordinate covalent bond is also known as a dative bond. In this chemical bond, both electrons are supplied by one of the two bonded atoms. This is commonly observed among coordinate covalent compounds and inorganic complexes and is written like a covalent bond.
How To Name Compounds and Write Compound Formulas
There are different types of compounds, but in this part, we will only consider the nomenclature of binary covalent and ionic compounds, as well as some inorganic acids.
There are certain rules to follow in naming binary compounds, and the figure presented below is an excellent guide.
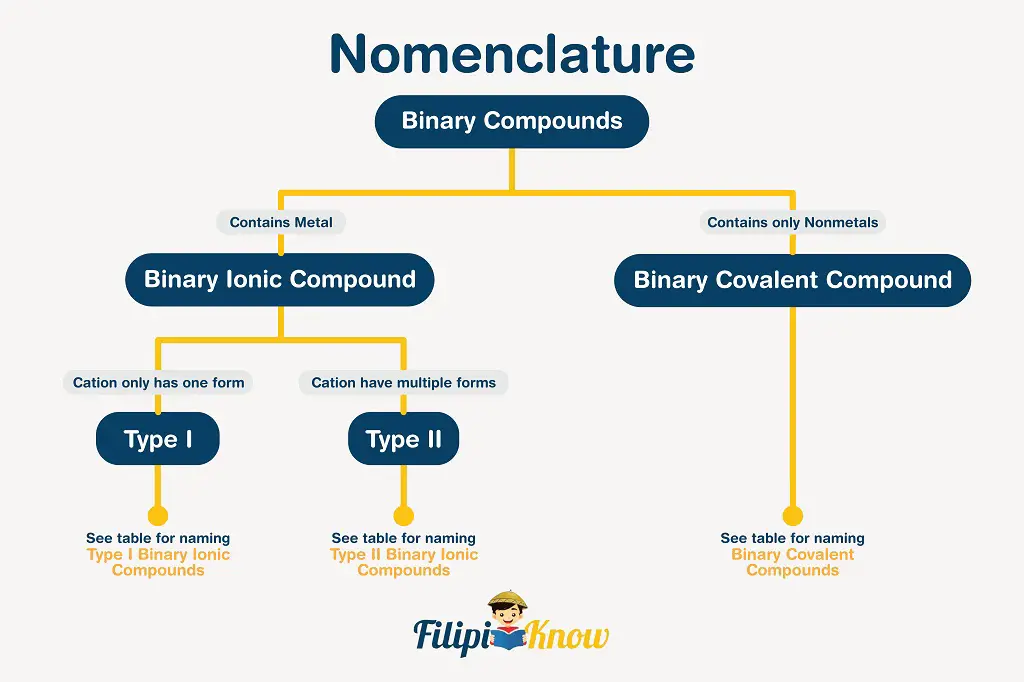
1. Binary Covalent Compounds
A binary covalent compound is a covalent compound that is made up of exactly two elements only.
a. Naming Binary Covalent Compounds
Rule 1: | The first element in the chemical formula is named first using the name of that element. |
Rule 2: | The second element in the chemical formula is named as if it is an anion. |
Rule 3: | Indicate the number of that element using the appropriate prefix. |
Rule 4: | The prefix mono– is never used in denoting the number of the first element. |
Rule 5: | If the name of the element starts with a vowel, drop the “a” or “o” in the prefix. |
The following prefixes must be used to denote the number of certain atoms in the compound’s formula.
Number | Prefix | Number | Prefix |
1 | mono- | 6 | hexa- |
2 | di- | 7 | hepta- |
3 | tri- | 8 | octa- |
4 | tetra- | 9 | nona- |
5 | penta- | 10 | deca- |
Examples:
Chemical Formula | Chemical Name |
N2O5 | dinitrogen pentoxide, not dinitrogen pentaoxide |
PCl5 | phosphorus pentachloride, not monophosphorus pentachloride |
CO | carbon monoxide, not monocarbon monooxide |
In N2O5, the name dinitrogen pentaoxide is incorrect by virtue of rule number 5, although if you will read older references, this system of nomenclature was allowed and accepted. However, the common practice today is to drop “a” or “o” in the prefix if the name of the element starts with a vowel. In PCl5, monophosphorus pentachloride is incorrect by virtue of rule 4. Lastly, for CO, monocarbon monooxide is incorrect by virtue of rules 4 and 5.
b. Writing Chemical Formula of Binary Covalent Compounds
Rule 1: | Identify the chemical symbol of the element. |
Rule 2: | Take note of the prefix used for each element, and write the corresponding number as a subscript of that element. |
Rule 3: | When writing the chemical formula, write the less electronegative element first. The most electronegative element in the periodic table is fluorine (F). Hence, you can make it a convention that the farther the element is from fluorine, the less electronegative it is. |
Examples:
Chemical Name | Chemical Formula |
oxygen difluoride | O is less electronegative than F; there is one O atom and two F atoms, hence the chemical formula is OF2, not F2O |
tetraphosphorus decoxide | P is less electronegative than F; there are four atoms of P and 10 atoms of O, hence the chemical formula is P4O10, not O10P4 |
sulfur hexaflouride | S is less electronegative than F; there is one S atom and 6 F atoms, hence the chemical formula is SF6, not F6S |
dioxygen difluoride | O is less electronegative than F; there are two O atoms and two F atoms, hence the chemical formula is O2F2, not F2O2 |
Always remember to write first the symbol for the less electronegative element in the chemical formula, followed by the more electronegative species. Fortunately, it is not a common mistake since most of the time, the less electronegative element is written first in the chemical name, so you can use it as a guide.
2. Binary Ionic Compounds
A binary ionic compound is a compound that is composed of a single type of cation and a single type of anion.
a. Naming Binary Ionic Compounds
Naming binary ionic compounds is a little more complicated than the nomenclature of binary covalent compounds due to the presence of cations with multiple oxidation states. If the cation exhibits only one oxidation state, then the compound is a Type I binary ionic compound. In contrast, if the cation exhibits at least two oxidation states, the compound is a Type II binary ionic compound.
i. Type I Binary Ionic Compounds
Rule 1: | The cation is always named first, while the anion is always named second. |
Rule 2: | Monoatomic cations are named as if they are elements. Meanwhile, the monoatomic anion takes the root of the element name + –ide. |
Rule 3: | Polyatomic cations and anions have special and unique names, and you should familiarize yourself with them. |
Rule 4: | The prefixes used in the nomenclature of covalent compounds are not usually used in the nomenclature of ionic compounds. |
The table below provides the list of the most common polyatomic ions you can encounter. It will be hard to remember them all at once, so it is suggested that you familiarize yourself with them by reading them repeatedly every day (familiarization is the key!).
Ion | Name | Ion | Name |
NH4+ | ammonium | SO42– | sulfate |
–OH | hydroxide | HSO4– | hydrogen sulfate/bisulfate |
C2H3O2– | acetate | SO32– | sulfite |
NO3– | nitrate | HSO3– | hydrogen sulfite/bisulfite |
NO2– | nitrite | CO32– | carbonate |
CN– | cyanide | HCO3– | hydrogen carbonate/bicarbonate |
MnO4– | permanganate | CrO42– | chromate |
O22– | peroxide | Cr2O72– | dichromate |
S2O32– | thiosulfate | AsO43– | arsenate |
ClO– | hypochlorite | PO33– | phosphite |
ClO2– | chlorite | PO43– | phosphate |
ClO3– | chlorate | HPO42– | hydrogen phosphate/biphosphate |
ClO4– | perchlorate | H2PO4– | dihydrogen phosphate |
Examples:
Chemical Formula | Chemical Name |
NaCl | sodium chloride |
NH4Br | ammonium bromide |
Ba(OH)2 | barium hydroxide, not barium dihydroxide |
(NH4)2SO4 | ammonium sulfate, not diammonium sulfate |
One of the most common mistakes committed when naming ionic compounds is the use of prefixes used for naming binary covalent compounds. Keep in mind that those prefixes are NEVER used when naming ionic compounds. Hence, the correct name of Ba(OH)2 is barium hydroxide and not barium dihydroxide. The same is true for naming (NH4)2SO4.
ii. Type II Binary Ionic Compounds
Rule 1: | The cation is always named first, while the anion is always named second. |
Rule 2: | In naming the cation using the systematic method, identify the oxidation state of the cation, and write the oxidation state (in roman numeral) adjacent to the name of the cation |
Rule 3: | In naming the cation using the stock system, identify the oxidation state of the cation. If the oxidation state of the cation is less than the other oxidation state, the cation is named as the element root name + –ous. On the other hand, if the oxidation state of the cation is greater than the other oxidation state, the cation is named as the element root name + –ic. |
Rule 4: | Name the monoatomic anion by taking the root of the element name + –ide. Use corresponding names for polyatomic anions. |
Rule 5: | The prefixes used in the nomenclature of covalent compounds are not usually used in the nomenclature of ionic compounds. |
As you can see, it’s getting more complicated as we proceed. First, you were asked to familiarize the prefixes, then the polyatomic ions, and now, the stock and systematic names! It is challenging, but again, familiarization is the key!
Metal ion | Stock Name | Systematic Name |
Pb2+ | plumbous | lead(II) |
Pb4+ | plumbic | lead(IV) |
Fe2+ | ferrous | iron(II) |
Fe3+ | ferric | iron(III) |
Co2+ | cobaltous | cobalt(II) |
Co3+ | cobaltic | cobalt(III) |
Cu+ | cuprous | copper(I) |
Cu2+ | cupric | copper(II) |
Examples:
Compound Formula | Stock Name | Systematic Name |
CuCl2 | cupric chloride | copper(II) chloride |
FeSO4 | ferrous sulfate | iron(II) sulfate |
SnBr4 | stannic bromide | tin(IV) bromide |
Pb(OH)2 | plumbous hydroxide | lead(II) hydroxide |
Nowadays, stock names are less preferably used because of their ambiguity, specifically for cations with more than two oxidation states. For example, Mn can have oxidation states of +2, +3, +4, +6, and +7. The use of stock names for these cases makes the name confusing since it is not known which among the Mn2+, Mn3+, Mn4+, Mn6+, and Mn7+ is the manganous and manganic ion.
Let us now proceed with the nomenclature of inorganic acids. By definition, acids are compounds that release (directly or indirectly) free H3O+ in an aqueous solution. The schematic diagram shown below efficiently describes how the naming of inorganic acids is carried out.
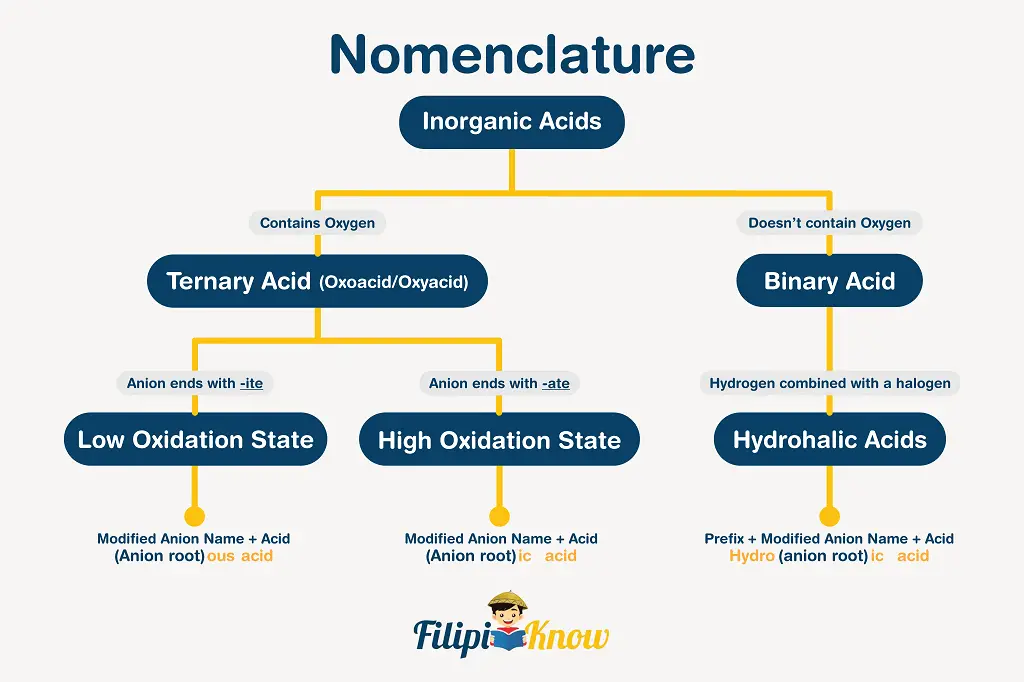
Examples:
Chemical Formula | Chemical Name |
HCl | there is no oxygen atom in the anion, hence the name is hydrochloric acid |
HBr | there is no oxygen atom in the anion, hence the name is hydrobromic acid |
HNO3 | there are oxygen atoms in the anion, and the anion name is nitrate, hence, the name of the acid is nitric acid |
H2SO4 | there are oxygen atoms in the anion, and the anion name is sulfate, hence, the name of the acid is sulfuric acid |
HNO2 | there are oxygen atoms in the anion, and the anion name is nitrite, hence, the name of the acid is nitrous acid |
H2SO3 | there are oxygen atoms in the anion, and the anion name is sulfite, hence, the name of the acid is sulfurous acid |
Additional polyatomic anions you can commonly encounter in the nomenclature of inorganic acids are shown below.
Formula | Name | Formula | Name | Formula | Name |
ClO– | hypochlorite | BrO– | hypobromite | IO– | hypoiodite |
ClO2– | chlorite | BrO2– | bromite | IO2– | iodite |
ClO3– | chlorate | BrO3– | bromate | IO3– | iodate |
ClO4– | perchlorate | BrO4– | perbromate | IO4– | periodate |
b. Writing Chemical Formula of Binary Ionic Compounds
Rule 1: | Separately write the cation and anion, indicating their correct charge. |
Rule 2: | Using the criss-cross method, take the absolute value of the charge of the cation, and write it as a subscript of the anion. In the same way, take the absolute value of the charge of the anion and write it as the subscript of the cation. |
Rule 3: | For polyatomic ions, write parenthesis if necessary. |
Rule 4: | The subscripts of the anion and cation must be written in the lowest possible terms. As an example, the formula of ferrous sulfate is written as FeSO4, instead of Fe2(SO4)2 since the subscripts of both ions are 2. In the same manner, the formula of tin(IV) sulfate is Sn(SO4)2 instead of Sn2(SO4)4. |
Examples:
Chemical Name | Ions involved | Chemical Formula |
calcium hydroxide | Ca2+, –OH | Ca(OH)2 |
ammonium chloride | NH4+, Cl– | NH4Cl |
barium phosphate | Ba2+, PO43– | Ba3(PO4)2 |
ferrous dichromate | Fe2+, Cr2O72– | FeCr2O7 |
lead(II) iodide | Pb2+, I– | PbI2 |
cupric nitrate | Cu2+, NO3– | Cu(NO3)2 |
The trick here that you need to remember is always to write the cation first (with its correct charge) followed by the anion (with its correct charge) like what was done in the second column.
Next, whatever the charge of the cation is, take its absolute value and make it the subscript of the anion. Then, take the absolute value of the charge of the anion and make it the subscript of the cation. Make sure to include parentheses if the ion is polyatomic! For example, in calcium hydroxide, there is a huge difference between Ca(OH)2 (the correct formula) and CaOH2 (non-existent).
Another thing that you need to remember is to always write the subscript of the chemical formula to the lowest possible terms. For example, by following the rules described for calcium hydroxide, the formula of ferrous dichromate should be (Fe)2(Cr2O7)2. However, due to this additional rule, the correct formula is FeCr2O7.
But before you write in the lowest possible terms, always make sure that you are dealing with an ionic compound, because this rule does not apply to binary covalent compounds. For example, P4O10 (a binary covalent compound) cannot be written as P2O5! Try to name these compounds, and you will see that these two are distinct from one another!
Determining Oxidation Number
An oxidation number is a number (positive, negative, or zero) assigned to a certain element to indicate its degree of oxidation or reduction. You will realize the importance of determining the oxidation number in the nomenclature of binary ionic compounds, and in balancing redox reactions.
Certain rules were established to allow us to determine the oxidation number of a certain element easily. This material will walk you through the step-by-step process (with illustration) of determining the oxidation numbers of a certain element in a compound.
Rules in Assigning Oxidation Numbers
Rule 1: | Free/uncombined elements have oxidation numbers equal to zero. Example: H2, Al, Fe, Zn, and N2 all have zero oxidation numbers. |
Rule 2: | Monoatomic ions have oxidation numbers equal to their charge. Example: The oxidation number of Fe2+ is +2, and the oxidation number of Cl– is -1. |
Rule 3: | In its ionic form, the oxidation number of Group I elements (except H) is always +1, while the oxidation number of Group II elements is always +2. |
Rule 4: | The oxidation number of H is +1. However, when combined with metal in hydride compounds, its oxidation number is -1. Example: In lithium hydride, LiH, the oxidation number of H is -1. |
Rule 5: | In all of its compounds, Al has an oxidation number of +3. Also, F has an oxidation number of -1 in all of its compounds. |
Rule 6: | The oxidation number of oxygen is -2, except for hydrogen peroxide (H2O2) or peroxide anion (O22–), wherein its oxidation number is -1. |
Rule 7: | Halogens have negative oxidation numbers when it forms halide compounds. However, when it forms oxygen-containing compounds, its oxidation number is positive. |
Rule 8: | For polyatomic ions, the algebraic sum of the oxidation number of each element is equal to the charge of the ion. |
Rule 9: | In neutral compounds, the algebraic sum of the oxidation numbers of all the elements is equal to zero. |
Sample Problems:
Determine the oxidation number of highlighted atoms in the following species:
- He
- KMnO4
- Cr2O72–
- C6H12O6
Solutions:
1. Helium (He) is a free element, therefore, by virtue of rule 1, its oxidation number is zero.
2. In KMnO4, the K is a Group 1 cation, and its oxidation number is +1 by virtue of rule 3. On the other hand, O has an oxidation number of -2 by virtue of rule 6, but since there are four oxygen atoms, each has an oxidation number of -2, then the total oxidation number contributed by the oxidation atoms is (4)(-2) = -8.
Finally, since KMnO4 is a neutral compound, the algebraic sum of the oxidation numbers of K, Mn, and O must be zero in accordance with rule 9.
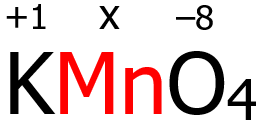
(+1) + x + (-8) = 0
x = -1 + 8 = +7
Therefore, the oxidation number of Mn is +7.
3. By virtue of rule 6, O has an oxidation number of -2, but since there are 7 O atoms, then (7)(-2) = —14. By virtue of rule 8, the algebraic sum of the oxidation numbers of Cr and O should be equal to -2. Hence:
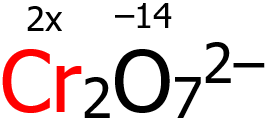
2x + (-14) = -2
2x = -2 + 14 = +12
x = +12⁄2 = +6
Why 2x, you ask? We are looking for the oxidation number of Cr, and it so happens that there are 2 Cr atoms in the chemical formula, hence 2x.
Based on the computation above, the oxidation number of Cr is +6.
4. By virtue of rule 6, the oxidation number of O is -2, hence (6)(-2) = -12. Furthermore, the oxidation number of H is +1 by virtue of rule 4, giving us (12)(+1) = +12. Finally, the algebraic sum of the oxidation numbers of C, H, and O must be equal to zero according to rule 9.
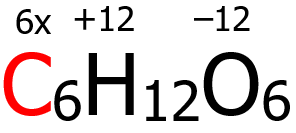
6x + (-12) + (+12) = 0
6x + 0 = 0
x = 0
Hence, the oxidation number of C is 0.
Representation of Compounds
As you may have noticed by now, the elemental composition of compounds is one of the information that can be obtained from its chemical formula. In water (H2O), for example, you can immediately say that per molecule of water, there are two atoms of hydrogen and an oxygen atom. This follows that in every mole of water, there are 2 moles of hydrogen and 1 mole of oxygen. This can then be expressed to mass to get the percent composition by mass.
Percent composition by mass is the percent by mass of each element in a compound. What is its importance, you ask?
Well, one of the most famous applications of this concept is the verification of a reagent’s purity. For example, a certain chemical manufacturing company claims that it can synthesize pure NH4NO3. One way chemists can verify this claim is to isolate one of the elements from the compound in its weighable form, then compare the experimental mass to the calculated mass. If the experimental data matches the calculated value, the manufacturer’s claim is true.
To compute the percent composition by mass of a certain element in the compound, the formula shown below can be used:

where n is the number of moles of the element per mole of the compound. Let us work with some examples to better understand this concept.
Sample Problem 1:
Calculate the weight percent of C (MM = 12 g/mol), H (MM = 1 g/mol), and O (MM = 16 g/mol) in C6H12O6 (MM = 180 g/mol).
Solution:
Solving for the percent composition of C (n = 6):
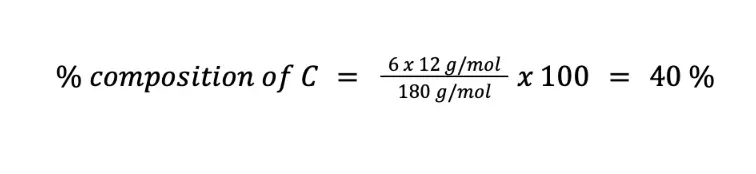
Meanwhile, the percentage composition of H (n = 12) is:
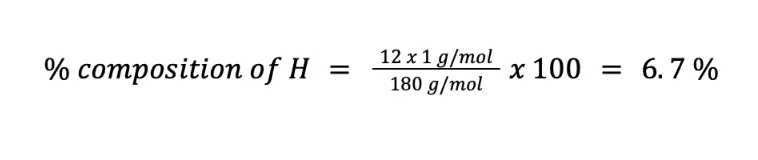
Finally, we can solve for the percent composition of O in two ways. First, you can solve by using the formula of the percent composition (n = 6):

Alternatively, since there are only C, H, and O in the chemical formula of C6H12O6, you can just subtract the sum of % C and % H to 100 %.

Sample Problem 2:
Ammonium nitrate (NH4NO3; MM = 80 g/mol) is widely used in agriculture as a nitrogen-rich fertilizer. How many grams of N (MM = 14 g/mol) is present in a 100g pure NH4NO3?
Solution:
Based on the chemical formula, we can readily say that there are 2 moles of N per mole of NH4NO3. This means that n = 2. Using the formula to calculate % composition of N by mass:
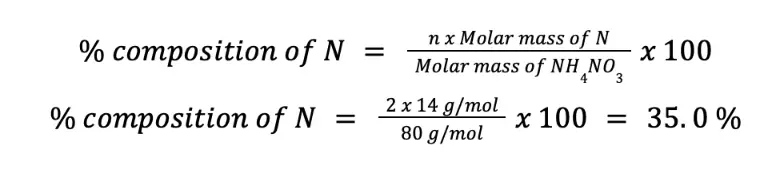
Since N is 35.0 % by mass with respect to NH4NO3, there are 35 g N per 100 g pure NH4NO3.
Writing Lewis Structure and Molecular Geometry
By definition, molecular geometry is the orientation of atoms of a molecule in space.
Is it really important to study the 3D structure of the compounds? Well, the answer is a big YES, since the compound’s geometry itself can directly affect the physical and chemical properties of a compound. This may include melting point, boiling point, density, and even reactivity.
For you to be able to draw correct molecular geometries, the most important prerequisite is you should know how to draw Lewis structures of chemical species. Lewis structures, also known as the electron dot structure, is a simple diagram showing the bond between two atoms, as well as the lone pairs around each atom in a chemical species.
Below is the set of rules you can use as a guide in writing the Lewis structures.
Steps in Writing Lewis Structures
Step 1: Identify the central atom. The central atom is usually the least electronegative atom among the atoms in the compound. The most electronegative element in the periodic table is fluorine (F). Hence, you can make it a convention that the farther the element is from fluorine, the less electronegative it is. However, H can never be the central atom, as H can only hold 1 covalent bond. Once you have identified the central atom, all the other atom(s) will be the substituent atom(s).
Step 2: Count the total number of valence electrons. The carbon group has 4 valence electrons, the nitrogen group has 5, the chalcogens (also known as the oxygen group) have 6, the halogen group has 7, and the noble gases have 8 valence electrons. In addition, hydrogen (H) has 1 valence electron, while beryllium (Be) has 2 valence electrons.
Step 3: Add the charge to the total number of electrons for negatively-charged species. Meanwhile, subtract the charge from the total number of electrons for positively-charged species.
Step 4: Draw a single bond first between the central atom and the substituent atom(s). Each bond is counted as 2 electrons. After subtracting the bonding electrons from the total number of electrons, distribute the remaining electrons among the substituent atom(s) first until each atom is already surrounded by 8 electrons. This rule is known as the octet rule. C, N, and O are strict followers of the octet rule. Hence, when these atoms are the central atom, you should make sure that there are only 8 electrons around these atoms. There are some exceptions though, all of which will be discussed in the examples presented below. If there are still electrons remaining, they must be distributed to the central atom.
Step 5: For charged species, the structure must be enclosed in a square bracket, with its net charge written as a superscript.
Let us work with some examples to better understand these steps.
Sample Problem 1:
Draw the Lewis structure of phosphorus pentachloride, PCl5.
Solution:
Step 1: P is less electronegative than Cl since it is farther from F, hence P is the central atom and Cl is the substituent atom.
Step 2: P belongs to the nitrogen group, while Cl is a halogen. Hence:
- valence electrons from P: 1 x 5e— = 5e—
- valence electrons from Cl: 5 x 7e— = 35e—
- total number of valence e—: 5e— + 35e— = 40e—
Step 3: The species being considered is neutral, hence no electron will be added or subtracted.
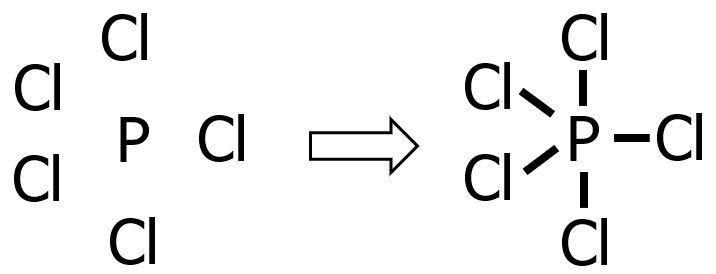
Step 4: Since we’ve already drawn 5 bonds, that means we already used 10 electrons (recall that each covalent bond consists of 2 e—) out of 40e—. Hence, we only need to distribute 30e—.
Distributing these 30e— to the substituent atom first until 8e— are present around each atom, we will have:
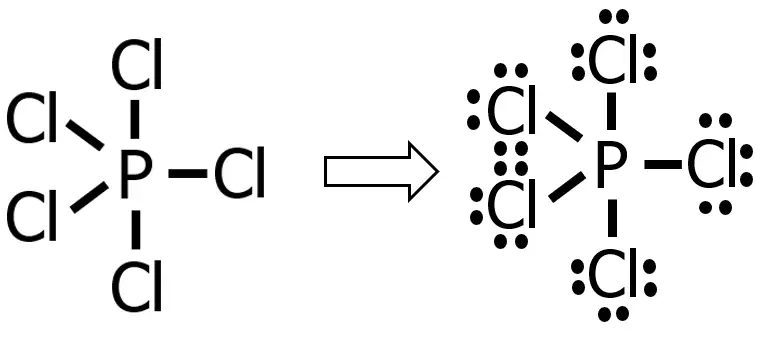
As you can see, we used up all the remaining 30e— so that there will be 8e— around each of the chlorine atoms. Since there are no more electrons to distribute, we’ve already drawn the Lewis structure of the compound. One thing you can notice in our Lewis structure is that the central atom does not follow the octet rule (notice that there are 10e— around the P atom). Such cases are known as an expanded octet. This usually occurs if the central atom is P, S, and the noble gases.
Sample Problem 2:
Draw the Lewis structure of ammonium ion, NH4+.
Solution:
Step 1: Since H can never be the central atom, then N will be the central atom of NH4+.
Step 2: N belongs to the nitrogen group, while H only has 1 valence electron. Hence:
- valence electrons from N: 1 x 5e— = 5e—
- valence electrons from H: 4 x 1e— = 4e—
- total number of valence e—: 5e— + 4e— = 9e—
Step 3: The species being considered is a cation with a +1 charge. Therefore, we need to subtract 1 from the total number of valence electrons, leaving us with 8e—.
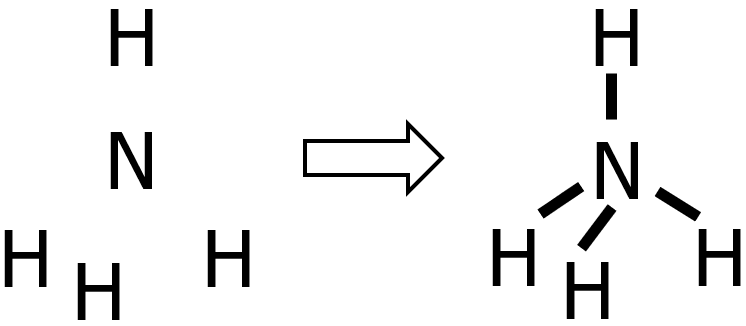
Step 4: Since we’ve already drawn 4 bonds, that means we already used 8e— out of 8e—. Therefore, there is nothing left to distribute. Take note that there are only 2e— around the H atoms, which is completely okay since H can hold up to 2e— only. Meanwhile, N has 8e— around it, so the octet rule was satisfied.
Step 5: Finally, since NH4+ is a cation, we need to enclose the Lewis structure inside a square bracket and write the charge as a superscript.
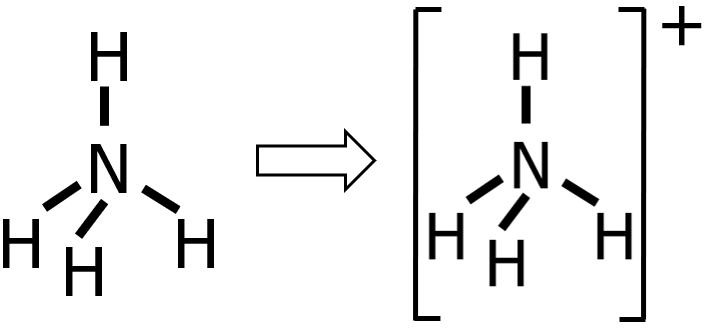
Aside from the expanded octet, another violation of the octet rule is the incomplete octet, commonly exhibited by beryllium compounds.
Try drawing the Lewis structure of BeCl2. You will notice that although the Cl atoms have 8e— around it, Be only has 4e—, hence, an incomplete octet. Aside from those, another violation of the octet rule is the duet rule, which is applicable only to hydrogen atoms since H can only hold a maximum of 2 electrons in its 1s orbital.
So far, we’ve only discussed single covalent bonds. How about double and triple bonds? How do we know if we need to draw multiple bonds? To answer this question, you will need to know the concept of a formal charge.
A formal charge is the electrical charge difference between the valence electron in an isolated atom and the number of electrons assigned to that atom in a Lewis structure. Mathematically, the formal charge can be determined using the formula:
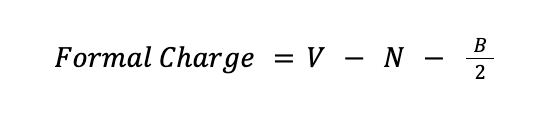
where V is the number of valence electrons of the atom, N is the number of nonbonding electrons around that atom in a Lewis structure, while B is the number of bonding electrons around the same atom in the Lewis structure.
Let us work with the sample problem below to better demonstrate how to determine formal charges and how it can be used to identify if multiple bonds are needed in a Lewis structure.
Sample Problem 3:
Draw the Lewis structure of sulfate ion, SO42—.
Solution:
Step 1: Since S is farther from F, S will be the central atom of SO42—.
Step 2: Both O and S are chalcogens, therefore:
- valence electrons from S: 1 x 6e— = 6e—
- valence electrons from O: 4 x 6e— = 24e—
- total number of valence e—: 6e— + 24e— = 30e—
Step 3: The species being considered is an anion with a charge of —2, therefore, we need to add 2e— to the total number of valence electrons, leaving us with 32e—.
Steps 4-5: Using the knowledge that we have so far, the Lewis structure of SO42— is shown in the figure below:
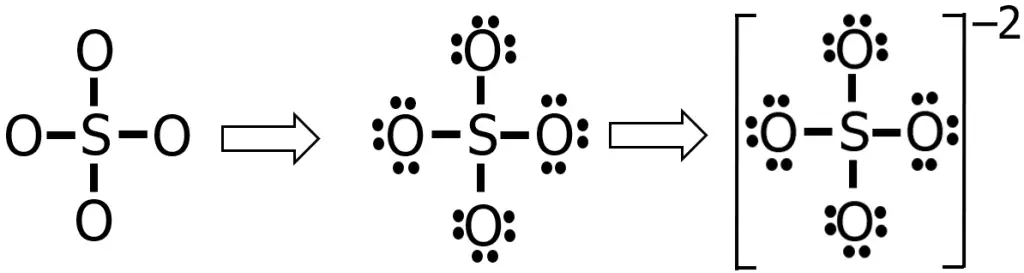
That seems good, right? All the electrons were distributed, and all the atoms obeyed the octet rule. However, you will find something unusual if you determine the formal charge of each atom in this Lewis structure.
To solve for the formal charges of each atom, it is worth noting that sulfur, being a chalcogen, has 6 valence electrons, hence V = 6. In the Lewis structure we currently have, there are no nonbonding electrons around the sulfur (N = 0), while there are 4 bonding electrons (B = 8). Hence, solving for the formal charge we have:

On the other hand, for oxygen, V = 6, N = 6, and B = 2. Therefore:

An ideal Lewis structure for any compound is a structure wherein the formal charge of all the atoms involved is, as much as possible, equal to or close to zero. So far, the formal charge of S in our current Lewis structure is +2, while all the oxygen atoms have a formal charge of -1.
Now, let me just do my stuff here, and let’s see what happens to the previous structure that we have.
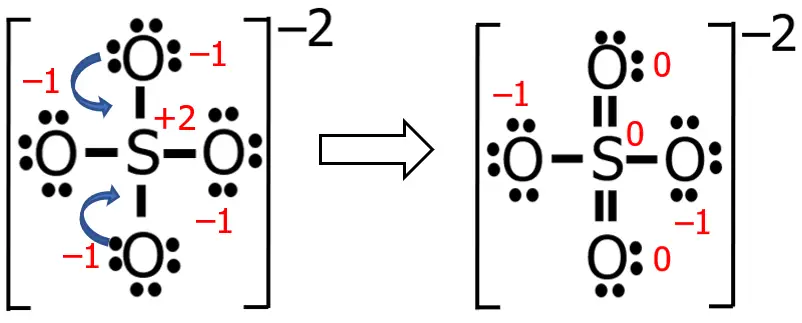
The numbers printed in red are the formal charges before and after the transformation. What did I do? Well, I just converted two nonbonding electron pairs (as the blue arrows suggest) into two bonding electron pairs. This makes the formal charges of three atoms zero (if you cannot believe it, you can solve it and see for yourself).
Hence, the more appropriate Lewis structure of SO42— is the latest version that we have. Take note that the algebraic sum of the formal charges is equal to zero for neutral molecules, while for ions, it should be equal to the charge of that ion.
TIPS: There is an easier way to determine the formal charge. You can think of an imaginary circle (or you can draw it if it is allowed to write on the questionnaire) around a certain atom, including all the lone pairs around it as well as the tip of the bond/s near it. Then, count the number of nonbonding electrons + bonding electrons around it, wherein each tip of the bond is counted as 1 electron only. Subtract this from the number of valence electrons of that atom, and you can immediately obtain the formal charge.
Try to determine the formal charge of the atoms in our previous examples and check if we draw the Lewis structure correctly!
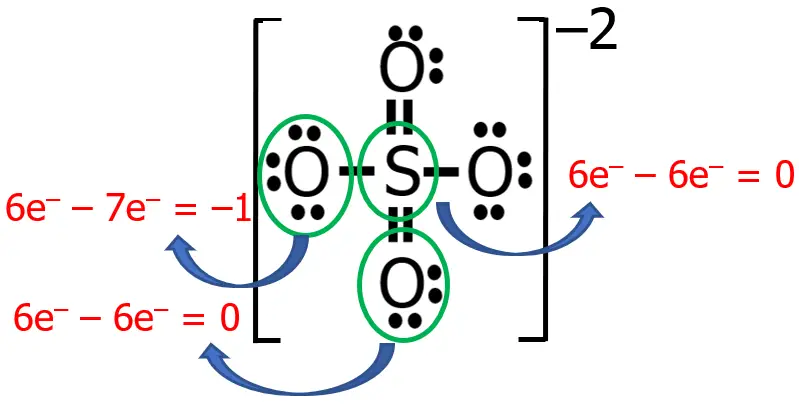
Now that you know how to draw the Lewis structure, it’s time to level up the structure from Lewis structure to molecular geometry.
In a Lewis structure, the orientation of atoms in space is not considered, making this structure a planar structure. However, not all molecules are flat. You might be asking yourself “If molecules have 3D structures, how are we supposed to write them on flat paper?” Good thing for us, there is what we call a solid wedge and a dashed (or broken) wedge.
A solid wedge denotes a covalent bond towards the viewer. You can also visualize it as if the atom on the other side of the solid wedge pops out of the paper. A dashed wedge represents a bond directed away from the viewer. Solid lines, on the other hand, represent a bond lying flat on the paper.
![]() | ![]() |
Molecular geometry of CH4 | Ball and Stick model |
In the structure of carbon tetrahydride above, more popularly known as methane, the hydrogens labeled 1 and 2 are on the surface of the paper, H3 is directed towards you, while H4 is directed away from you. Molecules assume geometries like this because they orient the substituent atoms in such a way that the distance between the two adjacent atoms is as far as possible.
Recall the bonding pairs that form the covalent bond are made up of electrons. To minimize the repulsive forces between these bonding pairs, the bonds must be oriented as far as possible. This approach to the study of molecular geometry is called the valence-shell electron-pair repulsion (VSEPR) model.
Shown in the table below are the most common electron pair geometries. For simplicity, we will just consider molecules of the form AXmEn, where A is the central atom, m is the steric number or the number of substituent atom X, and n is the number of lone pairs (E) on the central atom.

Take note that lone pairs are not drawn with a solid line and solid and broken wedges. The figure above presented it just to show that lone pairs assume a position that will maximize the distance between bonding and nonbonding electrons.
Let us work with some examples to better demonstrate this concept.
Sample Problem 4:
Identify and draw the molecular geometry of ClF3.
Solution:
Step 1: Without a doubt, Cl will be our central atom since F is the most electronegative element in the periodic table.
Step 2: Both Cl and F are halogens, therefore:
- valence electrons from Cl: 1 x 7e— = 7e—
- valence electrons from F: 3 x 7e— = 21e—
- total number of valence e—: 7e— + 21e— = 28e—
Step 3: The species being considered is a neutral compound. Therefore, no electrons will be added or subtracted, leaving us with 28e—.
Steps 4-5: Using the knowledge that we have so far, you know that there will be two lone pairs on the Cl atom for us to be able to distribute all 28e—. Furthermore, upon determination of formal charges, you will realize that no multiple bonds are necessary.
Hence, considering that the type of molecule is AX3E2, the molecular geometry of ClF3 is T-shaped.
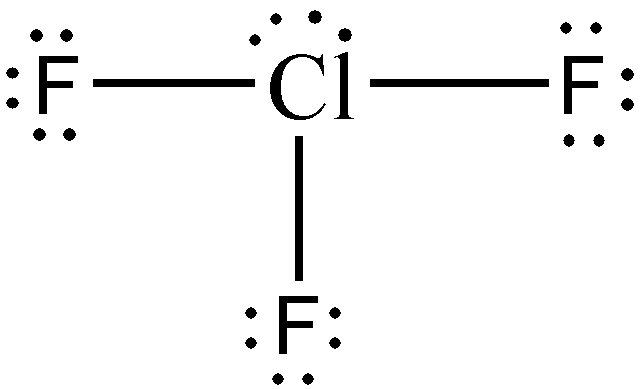
Take note that the two pairs of nonbonding electrons were not drawn with solid wedge and broken wedge, although it should be apparent that to minimize repulsive forces, one lone pair is situated somewhat towards you, while the other lone pair is directed away from you.
Additional Information: Electron Pair Geometry
In determining molecular geometry, we treat the lone pairs around the central atom as if they are not there. However, a type of chemical geometry treats the lone pair as if it is a substituent atom. This is the electron pair geometry. With this, ClF3 has a molecular geometry of T-shaped, but its electron pair geometry is trigonal bipyramidal.
Sample Problem 5:
Identify and draw the molecular geometry of PCl5 and SO42—.
Solution:
We’ve already constructed the Lewis structure of PCl5 from the previous sample problem (i.e., Sample Problem 1). From there, we have determined that PCl5 is an AX5 type of molecule. Hence, its molecular geometry is trigonal bipyramidal, and should be drawn as follows:
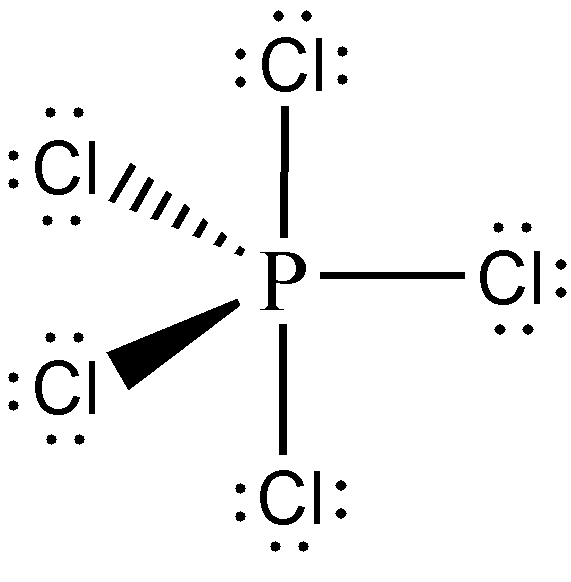
Meanwhile, the Lewis structure of SO42— was identified in Sample Problem 3, and we know that SO42— is an AX4 type of molecule. Therefore, its molecular geometry is tetrahedral, and should be drawn as follows:
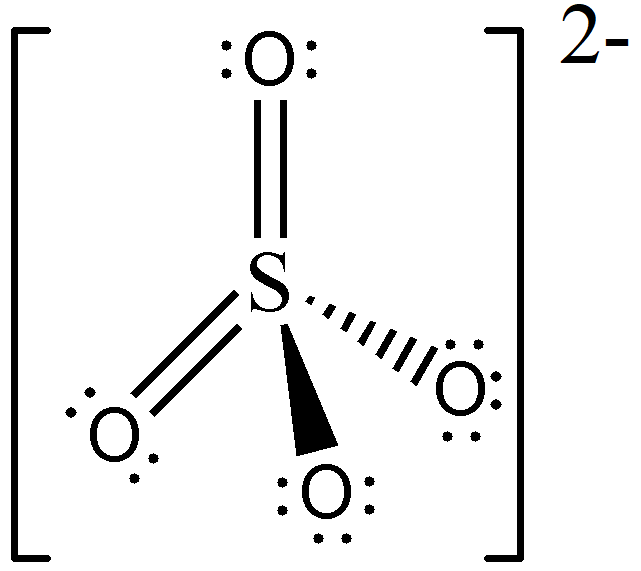
Take note that there is no way to draw multiple bonds using solid and broken wedges; hence, you should orient your drawing such that the multiple bonds will lie on the plane of the paper, like what we did in this example.
Intermolecular Forces of Attraction
So far, we’ve only discussed the types of chemical bonds that hold two atoms together in a compound. Such forces of attraction are called intramolecular forces (intra- means within). In this section, we will discuss the forces between molecules, also known as the intermolecular forces of attraction, commonly abbreviated as IMFA (inter- means between).
You might ask again, “Is it really important to study the forces that hold two molecules together?” Well for starters, some physical properties of compounds (i.e., melting point, boiling point, vapor pressure, etc.) can be explained largely by the IMFA that these compounds exhibit. For example, why does CCl4 (BP: 76.72 ℃) with a molar mass of almost 9x that of water (BP: 100 ℃) boil at a lower temperature? IMFA can explain that.
In general, there are three types of IMFA, namely: London dispersion Forces (LDF), dipole-dipole forces (DDF), and hydrogen bonding (H-Bonding).
London dispersion forces are a type of IMFA present in all substances, regardless of type, polarity, molar mass, and the like. It is also the weakest type of IMFA, and its occurrence is attributed to the fact that all substances, even the nonpolar ones, exhibit dipole moments momentarily.
By definition, the dipole moment is the product of charge and the distance between the charges in a molecule. We will not quantify dipole moments in this material, but you need to know that dipole moments alone dictate whether a certain compound is polar or nonpolar.
Polar compounds have a net dipole moment, while nonpolar compounds have no net dipole moment. As a result, it is very crucial that you know how to determine if a compound has a net dipole moment or none. One of the prerequisites to do so is that you should know how to draw its molecular geometry.
Let us work with some examples to demonstrate how to determine if a compound is polar or nonpolar using the net dipole moment.
Sample Problem 1:
Determine whether BeCl2 and H2O are polar or nonpolar.
Solution:
Using the knowledge that we have so far, we know that BeCl2 will exhibit linear geometry, whereas H2O will exhibit a V-shaped structure. We can determine if the compound is polar or not by drawing a dipole arrow (see figure below) and adding them vectorially. If all the dipole arrows cancel out, then the compound is nonpolar. Otherwise, it is polar.

Dipole arrows are drawn such that the head is pointing at the more electronegative atom. As you can see, adding the dipole moments of BeCl2 results in the cancellation of one another, which makes BeCl2 a nonpolar compound. Meanwhile, adding the dipole moments of H2O results in a net dipole moment that goes upward, hence water is a polar molecule.
Now, what do we mean by instantaneous dipole moments in LDF? Well, remember that covalent bonds are not rigid. This means that atoms can sway back and forth or up and down. In one specific instance, the bond of BeCl2 can look like what is shown below.
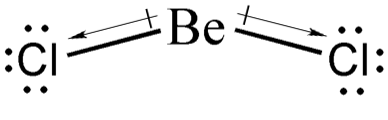
Notice that at that instant, BeCl2 has a momentary net dipole that points downward, giving rise to the London dispersion forces. Nonpolar compounds always have instantaneous dipole moments; however, these instantaneous dipoles cancel one another, making these compounds nonpolar.
Manual determination of polarity is tedious and time-consuming. To save time, you can use the table below as a guide. However, keep in mind that this is only applicable to compounds of type AXmEn, or those compounds with one type of substituent atoms only. If there is more than one type of substituent atom involved, then you have no choice but to determine the polarity manually.
Molecule Type/s | Molecular Geometry | Polarity | Examples |
AX2 | linear | nonpolar | BeCl2, HgCl2 |
AX3 | trigonal planar | nonpolar | BF3 |
AX2E, AX2E2 | bent/V-shaped | polar | H2O, SO2 |
AX4 | tetrahedral | nonpolar | CH4, NH4+ |
AX3E | trigonal pyramidal | polar | NH3, PCl3 |
AX5 | trigonal bipyramidal | nonpolar | PCl5 |
AX4E | seesaw | polar | SF4 |
AX3E2 | T-shaped | polar | ClF3 |
AX6 | octahedral | nonpolar | SF6 |
AX5E | Square pyramidal | polar | BrF5 |
AX4E2 | square planar | nonpolar | XeF4 |
The next type of IMFA is the dipole-dipole force (DDF), which exists only for polar substances. Collectively, LDF and DDF are known as van der Waals interactions.
DDF is a stronger type of IMFA relative to LDF. A special type of DDF is hydrogen bonding, exhibited only by compounds wherein a hydrogen atom is directly bonded to N, O, and F, although recent studies suggest that H2S also exhibits H-bonding.
How do we use the compound’s IMFA to predict some of its physical properties? Across compounds of relatively the same molar mass, stronger IMFA generally entails a higher boiling point and melting point, higher viscosity, higher surface tension, and lower vapor pressure.
Sample Problem 2:
Arrange the following set of compounds in order of increasing boiling point.
- F2 (MM = 38 g/mol), Br2 (MM = 160 g/mol), H2 (MM = 2 g/mol)
- Ne (MM = 20 g/mol), HCl (MM = 37 g/mol), H2O (MM = 18 g/mol)
Solution:
In (a), all compounds are linear and nonpolar, hence we can rank them in order of increasing boiling point based on their molar mass. In general, among compounds that exhibit the same type of IMFA, the higher the molar mass, the higher the boiling point. Hence, the correct sequence is H2 < F2 < Br2. True enough, H2 boils at –252.9 ℃, F2 boils at –188 ℃, and Br2 boils at 58.8 ℃.
Meanwhile, among compounds with relatively the same molar mass, the IMFA will dictate the trend in boiling point. Ne, being a nonpolar substance, exhibits only LDF. Meanwhile, HCl is a polar compound that exhibits both LDF and DDF, while H2O is also a polar compound that exhibits LDF, DDF, and hydrogen bonding. As a result, the correct sequence is Ne < HCl < H2O. This is supported by literature values since Ne boils at –246 ℃, HCl at –85.05 ℃, and water at 100 ℃.
References
Chang, R. (2010). Chemistry (10th ed.). New York City, USA: McGraw-Hill Companies, Inc.
Silberberg, M. (2006). Chemistry: The molecular nature of matter and change (4th ed.). New York City, USA: McGraw-Hill Companies, Inc.
Zumdahl, S., Zumdahl, S., & DeCoste, D. (2010). Chemistry (8th ed.). Belmont, California: Brooks Cole.
Next topic: Gases
Previous topic: The Periodic Table of Elements and Periodic Trends
Return to the main article: The Ultimate Chemistry Reviewer
Download Article in PDF Format
Test Yourself!
1. Practice Questions [PDF Download]
2. Answer Key [PDF Download]
Written by John Bryan Rolloque
in College Entrance Exam, LET, NMAT, Reviewers, UPCAT
John Bryan Rolloque
John Bryan Rolloque graduated cum laude at the University of the Philippines Los Baños in 2018 under the B.S. Agricultural Chemistry program. He taught courses in general chemistry, analytical chemistry, and organic chemistry at UPLB’s Institute of Chemistry, and has been serving as the Region IV coordinator for the Regional and National Chemistry Olympiad. Landing 8th place in the 2019 licensure exam for agriculturists, he is now taking up his master’s degree in plant physiology, also in UPLB.
Copyright Notice
All materials contained on this site are protected by the Republic of the Philippines copyright law and may not be reproduced, distributed, transmitted, displayed, published, or broadcast without the prior written permission of filipiknow.net or in the case of third party materials, the owner of that content. You may not alter or remove any trademark, copyright, or other notice from copies of the content. Be warned that we have already reported and helped terminate several websites and YouTube channels for blatantly stealing our content. If you wish to use filipiknow.net content for commercial purposes, such as for content syndication, etc., please contact us at legal(at)filipiknow(dot)net