The Cell
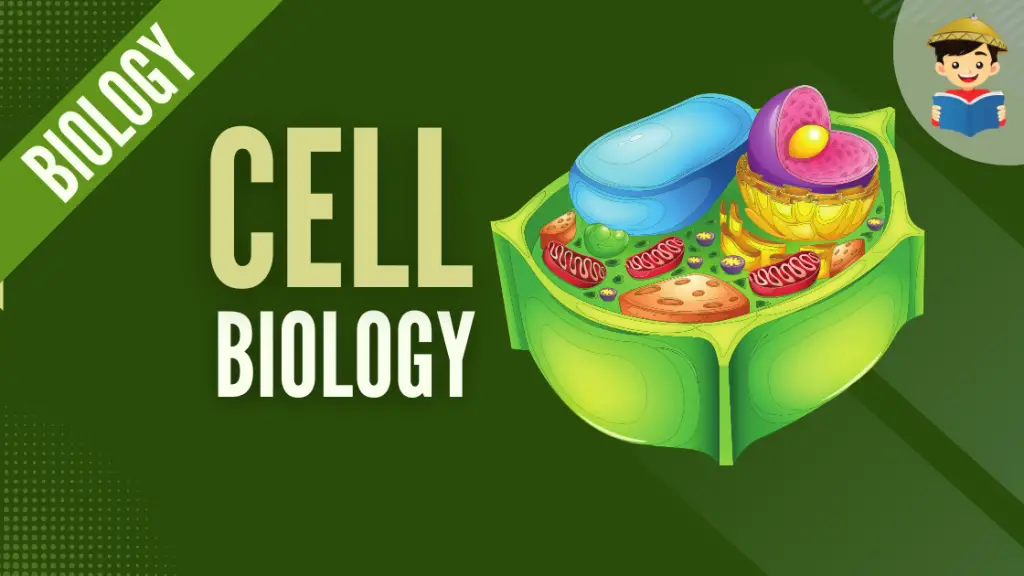
Every living being is composed of tiny structures called cells. In this reviewer, you’ll learn more about the structure and components of a cell, as well as different but important processes that occur in this microscopic object.
Click below to go to the main reviewers:
Table of Contents
- An Introduction to Cell Biology
- The Cell Theory
- Anatomy of the Cell
- Prokaryotes – Organisms with Simpler Cells
- Eukaryotes – Organisms with Complex Cells
- Animal and Plant Cells
- Cell Surfaces
- Cell Junctions
- Cell Transport Mechanisms
- Reproduction in Cells
- References
- Download Article in PDF Format.
- Test Yourself!
An Introduction to Cell Biology
The first time I held magnifying glass, I was amazed at how large things got from the other side of the glass. So you can only imagine my amusement when I was introduced to a microscope, an instrument with higher magnification, and told to look into a drop of water from a pond.
Discovering living organisms in pond water was what occurred with Anton van Leeuwenhoek, a Dutch scientist known for his works with microscopy and considered one of the pillars of microbiology. Although he did numerous microscope work with many microscopic organisms it was only later, when microscopes improved, when cells were first observed by English scientist Robert Hooke who also coined the term due to the appearance of what he saw as being those of a honeycomb shape.
The Cell Theory

Different microscopes have been developed over the years, and the most common you may have used in school laboratories are called light microscopes where light is passed through a specimen and then the microscope glass to magnify the image.
The aid from microscopes gave scientists evidence and helped our understanding of cells which piled up and points us to the concept of cell theory, the idea that living organisms are composed of basic, structural units called cells.
The theory believes in three main ideas:
- All living organisms are composed of one or many cells.
- Cells are the basic unit of structure and organization in organisms.
- Cells must come from pre-existing cells.
Advancements in technology and improvement in our understanding of the cell have also added the following additional concepts:
- DNA is passed between cells during cell division.
- All cells of organisms have the same basic chemical composition.
- Energy flow occurs within cells.
Anatomy of the Cell
Cells fall into one of two categories: prokaryotic and eukaryotic.
All cells share four common components:
- The plasma membrane – serves as an outer covering that protects the insides of a cell from its surrounding environment. It is usually composed of phospholipids arranged in a bilayer
- Cytosol – a fluid region in the cell where other cell components are found
- Chromosomes – structures that contain genetic material in the form of DNA
- Ribosomes – particles that synthesize proteins
The insides of both cell types are called the cytoplasm, but in eukaryotic cells, this refers to the region between the nucleus and the plasma membrane.
Because a eukaryotic cell is complex, it is often used to teach the parts of a cell. The following are components of the cell and the general functions they perform:
Parts | Characteristics/Functions |
Plasma Membrane | A semi-permeable layer that separates the insides of a cell from the external environment and is the location of chemical exchanges for many life functions. |
Cytoplasm | The fluid internal environment of the cell where all internal components of the cell (called “organelles”) are suspended. |
Nucleus | Stores the genetic material of the cell. In prokaryotes, this genetic material is located in a region called the nucleoid. |
Nuclear Membrane | Encloses the nucleus and protects the genetic material. |
Nucleoplasm | The environment within the nucleus. |
Nucleolus | An area in the nucleoplasm where the genetic material is highly concentrated. |
Nuclear Pore | Gateway of materials into or out of the nucleus. |
Mitochondrion | Produces energy for the organism, having the moniker “powerhouse of the cell” due to this function. |
Endoplasmic Reticulum (ER) | Sites for lipid and protein synthesis. |
Rough ER | Contains ribosomes and is the site for protein synthesis. In addition, it also functions as the cell’s membrane-making machine. |
Smooth ER | Contains cell types used in metabolic processes, especially lipid synthesis, as well as storage of calcium ions. |
Golgi Apparatus | Site for modifying, sorting, and storing compounds synthesized by the ER. |
Lysosome | Contains digestive enzymes that help break down food or damaged organelles. |
Peroxisome | Helps break down fatty acids to be used as cellular fuel. |
Ribosome | Sites of protein synthesis. Can be found attached to the rough ER or free in the cytosol. |
Vacuoles | Storage of food and other chemicals. |
Centriole | Organelle for cell division. |
There are also cellular parts that are present in specific groups of organisms, and there are also additional components of a cell that serve special functions in others. For example, chloroplasts are present in plants and used in photosynthesis.
The listed components are what is commonly observed in a typical eukaryotic cell. Earlier, it was mentioned that cells fall into categories. When these cell types compose an organism, they form the basis of whether an organism is considered a prokaryote or a eukaryote. A topic we will discuss next.
Prokaryotes – Organisms with Simpler Cells
Prokaryotes refer to organisms with cells that are simple, often single (unicellular), and lack a nucleus, or any other membrane-bound organelle. We will shortly come to see that this is significantly different in eukaryotes.
Prokaryotic DNA is found in the central part of the cell: a darkened region called the nucleoid.

Bacteria are considered prokaryotes. They have a cell wall made of peptidoglycan, composed of sugars and amino acids, and many have a polysaccharide capsule. This cell wall acts as an extra layer of protection, helping the cell maintain its shape, and preventing dehydration.
The capsule allows the cell to attach to surfaces in its environment. Other prokaryotes have flagella, pili, or fimbriae. Flagella are used for locomotion, while most pili are used to exchange genetic material during a type of reproduction called conjugation.
Eukaryotes – Organisms with Complex Cells
A eukaryote, meanwhile, is composed of cells that have a membrane-bound nucleus and other membrane-bound compartments, called organelles, with specialized functions.
The word eukaryotic means “true nucleus,” due to the presence of a membrane-bound nucleus in these cells. The word “organelle” means “little organ,” and organelles have specialized functions, similar to how our organs have specific functions.

In nature, form and function are apparent at all levels, including the level of the cell, and this will become clear as we explore eukaryotic cells.
The principle “form follows function” is found in many contexts. It means that, in general, one can deduce the function of a structure by looking at its form because the two are related. For example, birds and fish have streamlined bodies that allow them to move quickly through where they live, be it air or water.
Animal and Plant Cells
All eukaryotes–from protists (a diverse group of usually unicellular organisms) and fungi to animals and plants–are fundamentally similar. We will look into animal and plant cells as representatives of the eukaryotes.
The next two images are diagrams of a typical animal and plant cell.


The most obvious mark of a eukaryotic cell is its nucleus. But there are other organelles that perform specific tasks which can then be organized into the following basic functional groups:
- Genetic control, through the nucleus and ribosomes.
- Manufacture, distribution, and breakdown of molecules which include the endoplasmic reticulum, Golgi apparatus, lysosomes, vacuoles, and peroxisomes.
- Energy processing includes the mitochondria in all cells and chloroplasts in plants.
- Structural support, movement, and communication between cells are performed by the cytoskeleton, plasma membrane, and plant cell wall.
Except for lysosomes (which are not commonly found in plant cells) and centrosomes (organelles containing the centrioles), the organelles in animal cells are also found in plant cells.
Certain structures present in plant cells that animal cells lack are rigid and thick cell walls. This cell wall is made of cellulose which makes it chemically different from the cell wall of a prokaryotic cell.
Unique structures called plasmodesmata (singular plasmodesma) connect adjacent plant cells. Another key difference in plant cells is the chloroplast, where photosynthesis occurs. Plant cells also have a large, central compartment called a vacuole that stores water and a variety of chemicals.
Eukaryotic cells also contain non-membranous structures as well, of particular note is the cytoskeleton which is composed of different protein fibers that extend throughout the cell. Another example is ribosomes which can be found free in the cytosol as well as attached to certain membranes.
Cells are unique among organisms–from the way their surfaces are structured to the way they interact with each other. Cell surfaces and junctions between cells will be the focus of our next article.
Cell Surfaces
Networks of protein fibers extend throughout the cell. Collectively called the cytoskeleton, these fibers provide structural support as well as movement.
Three main kinds of fibers make up the cytoskeleton. From thickest to thinnest, they are:
- Microtubules – straight and hollow tubes composed of proteins called tubulins. In animal cells, microtubules grow from the centrosome while plant cells have other means of synthesizing as they lack centrosomes.
- Intermediate filaments – found in cells of most animals, these reinforce cell shape and anchor some organelles. For example, the outer layer of our skin is made of dead skin cells composed of intermediate filaments.
- Microfilaments – also called actin filaments, help support the cell’s shape, particularly in animal cells that lack cell walls. Microfilaments are also involved in cell movements.

The role of the cytoskeleton in movement is observed in motile appendages that rise from certain cells. For example, short and numerous appendages called cilia propel the protist Paramecium. Other protists move using a flagellum, which is longer than the cilia but present in fewer numbers or as a sole structure per cell.
Cell Junctions
The plasma membrane is usually considered the boundary of the cell, but most cells secrete materials outside the plasma membrane.
Animal cells produce an extracellular matrix (ECM) that helps hold cells together and protects and supports the plasma membrane. The main components of the ECM are glycoproteins, proteins bonded with carbohydrates.
The ECM may attach to the cell through other glycoproteins that bind to membrane proteins called integrins. As their name implies, they function in integration, i.e., transmit signals between the ECM and cytoskeleton; communicating changes occurring within and outside the cell.
Neighboring animal cells often interact and communicate through specialized junctions between them. The following image shows the three main types of cell junctions:

- Tight junctions occur when the plasma membrane of neighboring cells is knit tightly together by proteins. They prevent the leakage of fluid across a layer of cells. For example, the food we eat and are within our digestive tract does not leak because of these cell junctions.
- Anchoring junctions fasten cells into strong sheets. These junctions are connected to the cytoplasm by intermediate filaments. These junctions are common in tissues subjected often to stretching such as our skin and muscle.
- Gap junctions, also called communicating junctions, allow small molecules to flow through protein-lined pores between cells. Gap junctions are common in babies in the womb since communication between the mother and the developing baby is necessary for the baby’s development.
Earlier, it was mentioned that the cell wall is one of the features distinguishing plant cells from animal cells. Plant cells initially lay down a relatively thin and flexible primary wall to allow the cell to continue to enlarge. Between adjacent cells is a sticky substance called pectin, gluing cells together. When the plant cell stops growing, it strengthens the wall. Some cells add a secondary wall next to the plasma membrane. For example, wood consists mainly of secondary walls strengthened by the rigid molecule, lignin.
Although cell walls are thick, they do not isolate plant cells from each other. The plasmodesmata allow water and other small molecules to freely move from cell to cell. These structures allow cells in plant tissues to share water, nourishment, and chemical messages.

Because materials need to be transferred within, between, and among cells; we will tackle different transport mechanisms the cell employs in the next topic.
Cell Transport Mechanisms
Transporting substances to where they are needed is an essential feature of life. The transport of substances can be categorized as being active or passive, depending on if they require energy or not, respectively.
1. Passive Transport
a. Simple Diffusion
Molecules contain thermal energy due to their constant movement. One type of passive transport mechanism as a result of this property is diffusion.
Diffusion is the tendency of particles to spread out into available spaces. Randomly moving molecules will diffuse through air and water–or into and out of the cell. This spread can be visualized when trying to make drinks from powdered formulas or when adding dye to the water.

Particles will always spread from areas of high to a low concentration until an equilibrium is achieved. This difference in concentration between spaces is called a concentration gradient. Because this is an innate property of molecules, cells do not need to expend energy. This movement is thus considered a type of passive transport.
One of the most important substances that cross cells by passive transport is water. Because cells still need to regulate the number of substances that enter or exit it, its membrane is semi-permeable, that is to say, it allows easier entry for some substances than others. When water diffuses through this semi-permeable membrane this special type of diffusion is called osmosis.
b. Osmosis
Osmosis is a special type of diffusion wherein water or other solvents diffuse through a semi-permeable membrane.
Although water is an essential component of life, its entry and exit in cells must still be regulated. The term tonicity refers to the ability of the surrounding solution to cause cells to lose or gain water. This mainly depends on the concentration of solutes outside the cell with respect to the concentration of solutes inside the cell.
The following image shows the tonicity of a red blood cell:

Because animal cells lack a cell wall, these cells react at extremely high or low concentrations of solutes relative to the cell.
When the animal cell is immersed in an isotonic solution, where the concentration of solutes is relatively equal to those of the outside environment, the cell is able to maintain its shape. This is why intravenous fluids administered to patients must be isotonic to the blood to prevent complications.
When an animal cell is placed in a hypotonic solution, the solute concentration of the environment is lower than that of the cell (more water outside), resulting in water entering the cell, which then leads to lysis or burst.
If the opposite situation occurs and the animal cell is placed in a hypertonic solution, water concentration in the cell is higher than in the outside environment. As water tends to do, it will leave the cell causing it to shrivel, a process called crenation, due to water loss. As such, animals must be able to prevent excessive uptake or loss of water and regulate solute concentrations in their body fluids. This control of water balance is called osmoregulation.

Water balance is somewhat different for organisms with cell walls (see above image). Plant cells, for example, when subjected to a hypotonic environment, become turgid (very firm) and is the normal state of plant cells. Although the cell still swells, the cell wall also exerts pressure on the cell, called turgor pressure, preventing the plant cell from bursting.
In an isotonic environment, plant cells are flaccid; in a hypertonic environment, plant cells are no different from animal cells in that they lose water, shrivel, and their plasma membrane pulls away from the cell wall. This process is called plasmolysis and causes plants to wilt due to how lethal it is to the cells. The importance of this explains why we add salt to preserve meat and other foods as the salt causes food-spoiling bacteria or fungi to plasmolyze and eventually die.
c. Facilitated Diffusion
The plasma membrane of cells is composed of a phospholipid bilayer (each comprised of a hydrophilic phosphate head and a hydrophobic lipid tail), and this combination means that hydrophilic molecules and ions require help to be transported through the hydrophobic layer.
This assisted transport is called facilitated diffusion and is a form of passive transport as the only driving force is the concentration gradient. Different transport proteins embedded in the plasma membrane facilitate this type of transport.

The previous image shows transport proteins on the cell’s plasma membrane.
One transport protein provides a channel for specific molecules or ions to pass through the membrane. Another type, called carrier proteins, binds its passenger, which causes the protein to change shape, releasing the transported molecule on the other side. In both cases, the transport protein helps specific substances diffuse across the membrane through a concentration gradient, thus requiring no energy.
Water will have difficulty passing through the membrane, but the presence of protein channels called aquaporins allows for very rapid diffusion of water into and out of the cell.
Some materials in the cell cannot innately move on their own, and this can be a problem for the cell. So, it needs to spend energy to transfer those substances.
2. Active Transport
Another mode of transport, called active transport, requires cells to expend energy for substances to be moved against a concentration gradient. Imagine how harder it is to swim against a current than going along with it, say when swimming in a river.
Active transport allows cells to maintain internal concentrations of small molecules and ions. Protein pumps are often used to push solutes against a concentration gradient. The process uses energy in the form of ATP to allow transport proteins to change shape in such a way that it is able to transfer substances on the side opposite the concentration gradient. Upon expending energy, the protein reverts to its original shape.
a. Endocytosis and Exocytosis
Smaller molecules are relatively easier to move across cell membranes. However, it is quite different when larger molecules or substances must enter or leave cells. Two processes are involved in such cases.
The first is exocytosis, wherein bulky materials such as proteins or polysaccharides must be exported out of the cell. The Golgi apparatus facilitates this movement in cells by creating transport vesicles that move to the edge of the cell, fusing with the plasma membrane and releasing its contents. For example, insulin in the pancreas is introduced into the blood through exocytosis.

The other type called endocytosis is the opposite where large molecules or droplets of fluid are taken into the cell.
There are three types of endocytosis:
- Phagocytosis occurs when a cell engulfs a particle by wrapping around it with extensions called pseudopodia and packaging it as vacuoles. The vacuole must then fuse with a lysosome to digest the contents of the vacuole.
- Receptor-mediated endocytosis allows cells to acquire specific substances. Receptors are proteins for specific molecules, and once they bind with a specific compound, they begin engulfing those substances.
- Pinocytosis allows the cell to engulf small particles of fluids.

Cells have limited lifespans, and in order to keep the organism alive, these cells need to proliferate. How cells do this will be our focus in the next topic.
Reproduction in Cells
The ability to reproduce is one of the key characteristics of life. People can make more people because each organism carries specific genetic information at the cellular level.
Remember how eukaryotes carry DNA in the nucleus? When a cell undergoes reproduction or cell division, the resulting “daughter” cells are genetically identical to their original “parent” cell. Cells duplicate their chromosomes, structures containing DNA, before splitting so that the daughter cells receive identical sets of genetic material as their parent.

Cell division sometimes results in the reproduction of an entire organism. Single-celled organisms usually reproduce by dividing in half, termed binary fission, resulting in offspring that are genetic replicas of the parent cells. This is an example of asexual reproduction as this does not involve sperm and an egg cell. In a way, organisms that reproduce asexually give rise to clones or genetically identical individuals.
Sexual reproduction is different as it requires the fusion of gametes, cells specialized for reproduction, i.e., egg and sperm cells. Gametes only have half the chromosomes as the parent, but they contain unique combinations of genes. Once they fuse with another compatible gamete, the combined chromosomes form a more unique individual. In contrast to asexual reproduction, offspring produced in this manner are not identical to their parents or each other, except in the case of identical twins, as they inherit unique combinations of genes from their two parents.
Eukaryotic cells, in general, are larger and more complex than prokaryotic cells. They also usually have many more genes that specify an organism’s inherited traits. Almost all genes are found in the cell nucleus, grouped into multiple chromosomes. Each chromosome contains one long DNA molecule and a number of protein molecules attached to the DNA. Together, this structure is called chromatin, a mass of long, thin fibers.
As a cell prepares to divide, the chromatin must coil into tight structures called chromosomes. Why must it be compact? Imagine when you have to move and live in a new place: your belongings are spread around your home, but when you move you have to gather and pack them up into containers to make them easier to transport.
The chromosomes of a eukaryotic cell are duplicated before they condense and the cell divides. Each chromosome contains two copies called sister chromatids, joined copies of the original chromosome held together by proteins. The two sister chromatids are attached by proteins to form a region called the centromere.
When the cell divides, the sister chromatids separate from each other and are considered individual chromosomes but identical to the original chromosome. During cell division, a chromosome goes to each daughter cell. In this way, each daughter cell receives a complete and identical set of chromosomes.
1. The Cell Cycle
Cell division is the basis of reproduction for every organism. It is a key component of the cell cycle, an ordered sequence of events that run from the moment a cell is formed until it divides into two cells.
Two main stages comprise the cycle: a growing stage, called interphase, where the cell doubles everything in its cytoplasm and replicates DNA, and the actual cell division stage, called the mitotic phase.
a. Interphase (The Cell Grows)
Most of the cell cycle is spent in the interphase. During this phase, the cell performs its usual function. Interphase is divided into three subphases:
i. G1 phase or the “first gap.”
ii. S phase (for “Synthesis” of DNA) where chromosomes are duplicated and DNA is copied.
iii. G2 phase or the “second gap” where the cell completes preparation for cell division.
Cells are still active throughout all three subphases of interphase, making the “gaps” a misnomer. An alternative would be to remember them as “growth” substages.
Buckle up as we now come to the point where we will examine a closer look at one of the two major cell division processes, mitosis.
b. Mitotic Phase (The Cell Divides)
The mitotic phase refers to the period during which a cell undergoes physical division and is divided into two overlapping stages: karyokinesis or mitosis and cytokinesis.
In karyokinesis, the nucleus and its contents (emphasis on the duplicated chromosomes) divide and are distributed into the nuclei of the two daughter cells.
Cytokinesis, which begins before the end of mitosis, causes the cell’s cytoplasm to divide into two. The combination of these two produces genetically identical daughter cells, and each daughter cell may then proceed through G1 and repeat the cycle.
Of important note is that mitosis is unique to eukaryotes and is considered an evolutionary solution to problems arising from asexual reproduction. For example, if a farm is composed of only a single variant of a crop, it is possible that a single disease will afflict all of them compared to when the farm is composed of different variants of the same crop. Think BT corn being used by farmers in our country alongside local corn variants.
Stages of Mitosis
Mitosis is a continuous process distinguished into four basic stages: prophase, metaphase, anaphase, and telophase. In other references, another stage occurs between prophase and metaphase called the “prometaphase.” What occurs in this stage is usually listed under prophase.
In this article, we will provide information on all five stages. The chromosomes are the stars of this mitotic “dance.” Their movements depend on mitotic spindle fibers which emerge from the centrosomes. In each of the stages, the following occurs to the cell:
Prophase
- Changes occur in both the nucleus and cytoplasm. In the nucleus, the chromatin forms chromosomes and begins to duplicate into sister chromatids. In the cytoplasm, microtubules grow from centrosomes forming mitotic spindles that begin to move away from each other.
Prometaphase
- The nuclear envelope dissolves. Microtubules extend into the nuclear region and reach the chromosomes. Each sister chromatid contains sites called kinetochores where some of the spindles attach. The spindles move chromosomes toward the center of the cell.
Metaphase
- The mitotic spindle is fully formed, with its poles at opposite ends of the cell. The chromosomes line up on an imaginary plate in the middle of the cell called the metaphase plate, with the centromeres lined up on the plate. For each chromosome, the kinetochores of the sister chromatids are attached to opposite spindles from each pole.
Anaphase
- The centromeres come apart, separating the sister chromatids towards opposite poles of the cell. As this happens, the spindle microtubules attached to the chromatids shorten while those unattached lengthen. This moves the poles further apart so that by the end of this stage, there are equal collections of chromosomes on the two poles.
Telophase
- The cell continues to elongate. Daughter nuclei appear, and the nuclear envelope begins to form around the chromosomes. In terms of process, this is like the reverse of what occurred in the prophase. By the end of this stage, the chromatin uncoils, and the mitotic spindle disappears.

Mitosis, the division of one nucleus into two genetically identical daughter nuclei, is finished. Cytokinesis, the division of the cytoplasm simultaneously occurs with telophase and differs between animal and plant cells.
In animal cells, cytokinesis occurs by cleavage, and it appears as a cleavage furrow, a shallow groove on the cell’s surface. This site has a microfilament ring that ultimately contracts and pinches the cell into two.

In the case of plant cells, the stiff cell wall prevents contraction. What happens is that during telophase, vesicles containing cell wall material collect in the middle of the parent cell, forming a disk called a cell plate. The cell plate grows outward, accumulating more cell wall material as vesicles fuse with it until it fuses with the plasma membrane and the cell plate joins the parental cell wall.
Mitosis is just one mechanism. The next mechanism we will discuss refers specifically to sex cells; the process known as meiosis.
2. Meiosis and Crossing Over
In eukaryotes, a typical body cell called a somatic cell has a distinct number of chromosomes. Humans, for example, have 46 chromosomes. Almost every chromosome has a twin that resembles it called homologous chromosomes (or homologous pair) because each chromosome carries genes controlling the same inherited characteristics.
The genes, structures that contain inheritable traits, for these characteristics or traits can be located at a particular place on the chromosome called a locus (plural loci). However, the two chromosomes have different versions of the same gene.
For example, one chromosome may have a gene that allows you to inherit freckles while the other chromosome has that gene at the same place, but it encodes in a way that you will not have freckles. The sequences of the two copies of the gene will ultimately affect the person’s appearance.

In humans, two distinct chromosomes, the X and Y chromosomes are exceptions to this general pattern of homologous chromosomes. Females have homologous pairs of X chromosomes (XX) while males have one X and one Y chromosome (XY).
Only small parts of X and Y are homologous with most genes on the X chromosome not appearing on the tiny Y, and the Y chromosome has genes not found in X. Because they determine an individual’s sex, these chromosomes are called sex chromosomes. Chromosomes other than these two are called autosomes.
Most animals and plants are diploid organisms, that is their somatic cells contain pairs of homologous chromosomes. The total number of chromosomes in an organism is called the diploid number (abbreviated 2n, this is equal to 46 in humans for example). The exceptions are the gametes as each contains a single set of chromosomes.
In our case from 46 total chromosomes, our gametes contain only 23 (remember 2n=46, so n=23) comprised of 22 autosomes plus either one of the sex chromosomes. These cells which contain a single member of the homologous pair are considered haploid cells. Sex cells are important for reproduction, and they are also created from a different form of cell division called meiosis, which occurs in our reproductive organs.
Stages of Meiosis
Meiosis is the cell division that produces haploid gametes in diploid organisms. Many of the stages of meiosis closely resemble corresponding stages in mitosis. Both are preceded by chromosome replication in interphase, but in meiosis, this duplication is followed by two consecutive cell divisions called meiosis I and meiosis II.
Because one duplication of chromosomes is followed by two divisions, this results in four daughter cells, each having half the number of chromosomes as the parent cell. We’ll take a closer look into the stages starting with meiosis I.
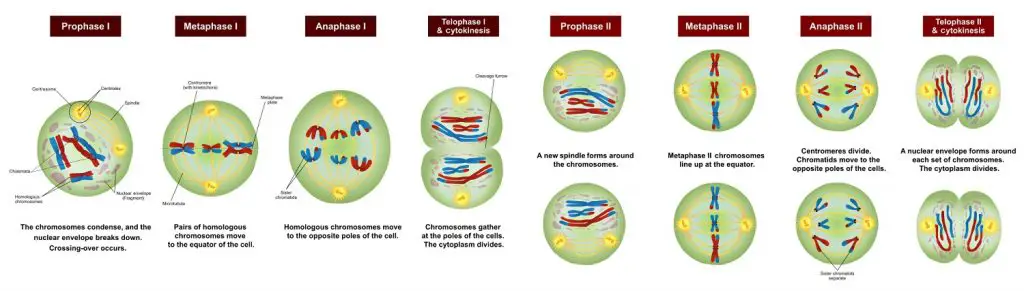
Meiosis I
Unlike mitosis which has the prometaphase stage, meiosis follows the four basic mitotic stages, accompanied by a Roman numeral to describe whether the sex cell is undergoing the first or second cell division.
Prophase I
- Homologous chromosomes, composed of two sister chromatids, come together in pairs forming a tetrad, four chromatids aligned gene by gene.
- Crossing over, one of the most important events in meiosis occurs, that is chromatids exchange segments with one chromatid of the other homologous pair. This rearranges genetic information.
- As prophase I continue, the chromosome coil, the nuclear envelope dissolves, and the tetrad is captured by the spindle microtubules and moves them to the center of the cell.
Metaphase I
- The tetrads are aligned on the metaphase plate. The spindles are attached to the kinetochores at the centromeres. In each tetrad, the homologous chromosomes are held together at the sites where crossing over took place.
- For each tetrad, the spindle from opposite poles of the cell attaches to one of the homologous pairs. This arrangement directs each tetrad to move toward opposite poles of the cell.
Anaphase I
- This stage is marked by the migration of chromosomes toward the poles of the cell.
- In contrast to mitosis, the sister chromatids remain attached, and only the tetrads which contain sites of cross-over split up.
Telophase I (and simultaneously Cytokinesis)
- The chromosomes arrive at the poles, resulting in each pole having a haploid set of chromosomes.
- After cytokinesis, two haploid daughter cells are formed.
In some organisms, interphase follows before meiosis II. In others, meiosis II ensues. In either case, no duplication of chromosomes occurs.
Meiosis II
Meiosis II can be summarized as sister chromatids separating and are essentially the same as mitosis. The difference is that meiosis II starts with a haploid cell. When the haploid sperm from the father fertilizes a haploid egg from a mother, the resulting fertilized egg called a zygote will contain one set of homologous chromosomes from each parent.
Cellular division is a highly regulated process. Cells in the human body, if left unchecked, may proliferate into an abnormal mass, forming tumors. Tumor cells, if left unregulated, may become lethal by becoming cancers in the body.
References
Alberts, B., Johnson, A., Lewis, J., Raff, M. C., Roberts, K., Walter, P., … Hunt, T. (2008). Molecular Biology of The Cell. New York: Garland Science.
Armstrong, P. (2007). All things Darwin: An encyclopedia of Darwin’s world. Westport, Connecticut: Greenwood.
Campbell, N. A., Cain, M. L., Minorsky, P. V., Reece, J. B., Urry, L. A., & Wasserman, S. A. (2018). Biology: A global approach. Harlow, Essex, England: Pearson Education Limited.
Chiras, D. D. (2008). Human Biology. Sudbury, Mass: Jones and Bartlett Publishers.
Fowler, S., Roush, R., & Wise, J. (2013). Concepts of Biology. Houston, Texas: OpenStax. Available at https://openstax.org/books/concepts-biology/pages/1-introduction.
Hine, R. (2019). A Dictionary of Biology. Oxford, US: Oxford University Press.
Hillis, D. M., Moritz, C., & Mable, B. K. (1996). Molecular systematics. Sunderland, Mass: Sinauer.
Hollingsworth, P. M., Bateman, R. M., Gornall, R. J., & Conference on Advances in Plant Molecular Systematics. (1999). Molecular systematics and plant evolution. New York: Taylor & Francis.
Jamora, C., Fuchs, E. (2002). Intercellular adhesion, signaling, and the cytoskeleton. In: Nature Cell Biology. Volume 4, E101–E108. Available at https://doi.org/10.1038/ncb0402-e101
Karp, G., Iwasa, J., & Marshall, W. (2018). Cell biology. Hoboken, NJ: John Wiley.
Lecuit, T., Pilot, F. (2003). Developmental control of cell morphogenesis: a focus on membrane growth. In: Nature Cell Biology. Volume 5, pages 103–108. Available at https://doi.org/10.1038/ncb0203-103
Lodish, H. F. (2013). Molecular Cell Biology. New York: W.H. Freeman and Co.
Meerman, R., & Brown, A. (2014). When somebody loses weight, where does the fat go? British Medical Journal. Vol. 2014. Issue 349.
Nelson, D. L., & Cox, M. M. (2017). Lehninger Principles of Biochemistry (7th ed.). W.H. Freeman.
Ochman, H. (2016). Microbial evolution: A subject collection from Cold Spring Harbor Perspectives in Biology. Cold Spring Harbor, New York: Cold Spring Harbor Laboratory Press.
Reece, J. B., & Campbell, N. A. (2011). Campbell Biology. Boston: Benjamin Cummings/Pearson.
Simpson, M. G. (2019). Plant systematics. Amsterdam Academic Press.
Taylor, M. R., Simon, E. J., Dickey, J., Hogan, K. A., & Reece, J. B. (2018). Campbell Biology: Concepts & connections. NY: Pearson.
Next topic: Cellular Metabolism
Previous topic: Biomolecules
Return to the main article: The Ultimate Biology Reviewer
Download Article in PDF Format.
Test Yourself!
1. Practice Questions [PDF Download]
2. Answer Key [PDF Download]
Written by Earl Jeroh Bacabac
in College Entrance Exam, LET, NMAT, Reviewers, UPCAT
Earl Jeroh Bacabac
Earl’s love for the sea fueled his goal to become a marine biologist. He obtained his Bachelor’s Degree in Biology from the University of the Philippines Visayas while also being a DOST scholar. His passion for the marine environment is rivaled by his diverse interests in music, the arts, and video games.
Copyright Notice
All materials contained on this site are protected by the Republic of the Philippines copyright law and may not be reproduced, distributed, transmitted, displayed, published, or broadcast without the prior written permission of filipiknow.net or in the case of third party materials, the owner of that content. You may not alter or remove any trademark, copyright, or other notice from copies of the content. Be warned that we have already reported and helped terminate several websites and YouTube channels for blatantly stealing our content. If you wish to use filipiknow.net content for commercial purposes, such as for content syndication, etc., please contact us at legal(at)filipiknow(dot)net