The Periodic Table of Elements and Periodic Trends
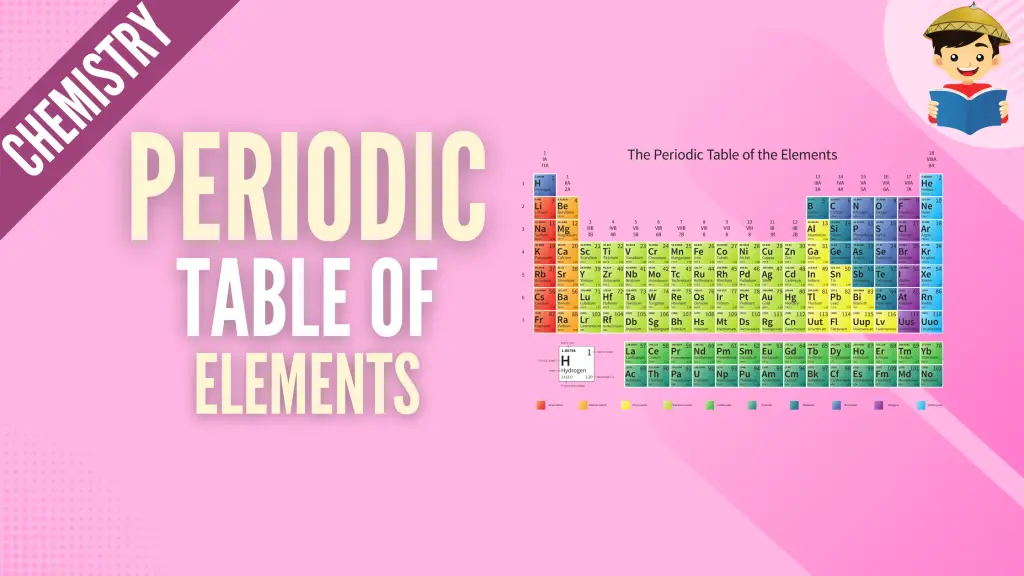
When you hear the word “Chemistry,” what comes to mind? There may be a lot of things, but one of those is probably the periodic table of the elements! In this chapter, we will talk about how elements are arranged in the modern periodic table and what important properties and trends we can obtain from this arrangement.
Click below to go to the main reviewers:
Table of Contents
- The Periodic Table of Elements
- Groups and Families in the Periodic Table
- Periodic Trends
- References
- Download Article in PDF Format
- Test Yourself!
The Periodic Table of Elements
The development of the periodic table as we know it today started in 1829 when Johann Dobereiner proposed the model of triads or the law of triads in some references. The model states that the atomic weight of the middle element in a set of three elements (he called triad) is approximately equal to the arithmetic mean of the two extreme elements in the set.
Dobereiner was able to identify several triads of elements with the same properties which adhere to this model. Unfortunately, it fails to apply to most of the elements known during Dobereiner’s time, proving the model severely lacking. Table 1 enumerates some of Dobereiner’s triads.
Element | Symbol | Atomic Mass |
Lithium | Li | 6.9 |
Sodium | Na | 23.0 |
Potassium | K | 39.0 |
Chlorine | Cl | 35.4 |
Bromine | Br | 79.9 |
Iodine | I | 126.9 |
Sulfur | S | 32.0 |
Selenium | Se | 79.0 |
Tellurium | Te | 128.0 |
Dobereiner’s model was followed by John Newland’s law of octaves in 1864. Newland observed that elements are arranged based on their atomic weights, elemental properties seem to repeat every eight elements. Although the law was able to group a few elements with similar properties successfully, it was generally considered unsuccessful.
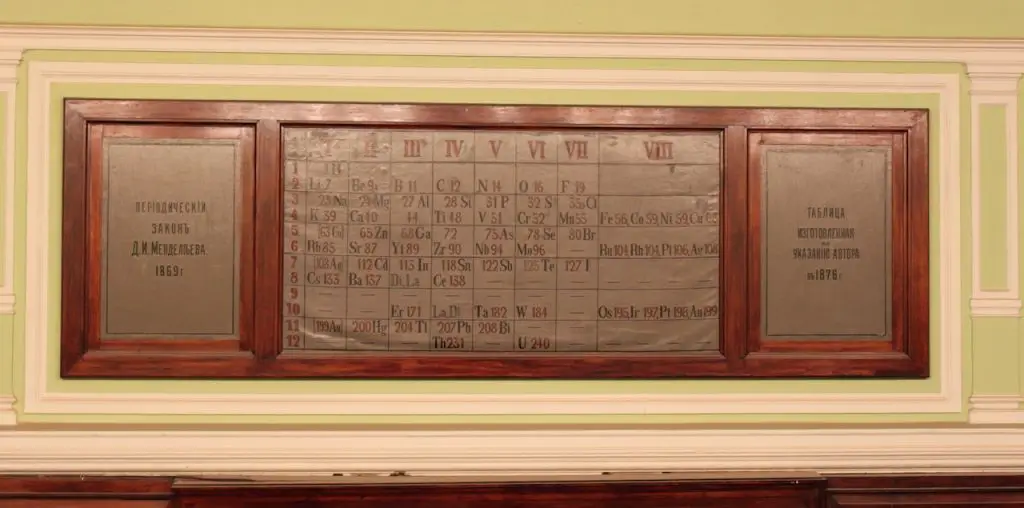
It was only during the time of Julius Lothar Meyer and Dmitri Ivanovich Mendeleev that the idea of a periodic table of elements was presented to the world. The two scientists independently formulated the table, but Mendeleev was given most of the credit because of his insistence on how important the table is.

Groups and Families in the Periodic Table
Unlike Mendeleev’s table which is arranged based on atomic mass, the modern periodic table that we know today is arranged based on atomic number. The rows in the table are known as periods, while the columns are known as groups or families. The table below shows the outline of the modern periodic table of elements.

Elements in groups 1, 2, and 3-17 are the representative elements or the main group elements. All the representative elements have their highest s or p orbital incompletely filled.
Belonging to the representative elements, group 1 is known as the alkali metals. Elements under this family are highly reactive and possess a single electron in their outermost shell. As a result, these elements readily lose one electron to form ions with a +1 charge. Group 2, on the other hand, is known as alkaline earth metals. Elements under this family have two electrons in their valence shell which they readily lose to form cations with +2 charges.
Together with the alkali metals, groups 1 and 2 (except H and Be) form strong inorganic bases when bonded with hydroxide ions. Furthermore, these two groups are sometimes referred to as the s block elements.
Group 18 (noble gases) have filled p subshells (except He). Aside from helium, all members of the group have 8 electrons in their valence shell. As a result, noble gases are chemically inert. They are also colorless, although ionizing them may cause these gases to emit bright colors. This is why noble gases (except Rn) are commonly used in neon signs.

Groups 3-12 comprise the transition metals. Elements belonging to these groups are generally characterized by partially filled d subshells or those which can give rise to cations with an incomplete d subshell. Because of this, transition metals are sometimes called the d block elements.
Some transition metals can form cations with multiple oxidation states. For example, chromium can have an oxidation state of either +3 or +6, while manganese can take +2, +3, +4, +6, and +7 as their oxidation states. Other transition metals capable of having multiple oxidation states are labeled in the modern periodic table of elements shown previously.
Group 13 is the Boron group (or icosagens), which all have three electrons in their outermost shell. This group forms cations with a +3 charge upon ionization.
Carbon group or crystallogens are those under group 14. Elements under this group are characterized by 4 electrons in their outermost shell.
Group 15 are the pnictogens, all of which have 5 valence electrons. Aside from nitrogen, pnictogens are solid at room temperature.
Chalcogens or the group 16 elements are composed of electronegative nonmetals and metalloids, all of which have 6 electrons in their outermost shell. Next to chalcogens are the halogens (Group 17), famously known as the only group in the periodic table with members existing as gas (fluorine and chlorine), liquid (bromine), and solid (iodine and astatine) at room temperature. Halogens have 7 electrons in their outermost shell; thus, these atoms readily accept an electron to form anions with a –1 charge.
Groups 13-18 are collectively known as the p block elements. The p block is made up of metals, nonmetals, and the so-called metalloids, or those elements with properties that are intermediate to both metals and nonmetals. B, Si, Ge, As, Sb, Te (enclosed in a square border in the outline of the modern periodic table of elements displayed earlier) are widely regarded as metalloids; Po and At are irregularly accepted; while Al and C are rarely recognized as metalloids. Because of their unique properties, metalloids are used in the manufacturing of semiconductors.
Aside from these families, there are also lanthanide and actinide series in the periodic table, collectively called the f block transition metals due to their incompletely filled f subshells.
Lanthanides are rare earth elements, as they are naturally occurring but exist in extremely small amounts (less than 0.001 % of the Earth’s crust). Estimates show that lanthanides are more abundant than other elements; however, they are highly dispersed in the Earth’s crust, making these elements “rare.” These elements have incompletely filled 4f subshells or those that form cations with incompletely filled 4f subshells.
On the other hand, actinides are all radioactive. They are heavy elements that are not naturally occurring, some are even named after famous scientists. Elements in the actinide series either have incompletely filled 5f subshells or form cations with incompletely filled 5f subshells.
Periodic Trends
Another benefit of using the periodic table of elements is the ease of determining trends in some of the properties relative to one another. In this topic, we will discuss trends in atomic radius, electronegativity, ionization energy, and electron affinity.
1. Atomic radius

Atomic radius is defined as one-half the distance between two adjacent atoms. Generally, atomic radius increases down the group and decreases across a period from left to right. This trend can be explained by the fact that as we go down the group, another energy level is added to the atom. Across a period, elements have the same energy levels. However, as we go from left to right, the number of electrons in the outermost shell increases. This increase in the number of electrons causes stronger interaction between the nucleus and the electrons in the valence shell. As a result, the atom becomes “compressed,” and the radius decreases.
2. Electronegativity

Electronegativity is the tendency of an atom to draw shared electrons in a chemical bond towards itself. The figure above shows the difference in electron density between bonded atoms in Cl2 (left) and HCl (right).
Because Cl atoms have the same electronegativity, you can see that the electron cloud in the two Cl atoms is uniformly distributed. However, in HCl, the difference in electronegativity between H and Cl causes electrons in the covalent bond to be drawn nearer to the Cl atom. As a result, the electron cloud is larger in the vicinity of chlorine compared to the vicinity of hydrogen.
Determining periodic trends in electronegativity is quite easy. You just need to remember that the most electronegative element in the periodic table is fluorine. Hence, electronegativity increases as you approach fluorine (i.e., electronegativity increases across a period from left to right and decreases down the group).
3. Ionization energy
Meanwhile, ionization energy is the minimum energy required (in kJ/mol) to remove one electron from an isolated atom or molecule.
One important thing to remember here is that the closer the electron to the nucleus, the stronger the attraction. Hence, electrons closer to the nucleus are harder to remove or in other words, have higher ionization energy. Removing a valence electron is relatively easier (since they are the one farthest from the nucleus) than removing one of the core electrons in an atom. Because of this, smaller atoms have higher ionization energy, and larger atoms have smaller ionization energy.
Therefore, ionization energy decreases down a group and increases across a period from left to right.
4. Electron affinity

On the other hand, electron affinity is the atom’s ability to accept one or more electrons. The more willing an atom is to accept an electron, the more positive its electron affinity is.
In the periodic table, the halogens or the group 17 elements have the most positive electron affinity. Therefore, electron affinity increases from left to right of the periodic table and decreases down the group.
This trend can also be related to the atomic radius of an atom. The electrons in the valence shell of smaller atoms are closer to the nucleus. As a result, valence electrons are held more tightly by the nucleus in smaller atoms. Upon entering, an incoming extra electron is stabilized by this stronger attraction with the nucleus. In contrast, an incoming electron into the valence shell of larger atoms is not stabilized due to weaker interaction between the nucleus and the electron. As a result, larger atoms have a lower affinity for electrons.
References
Chang, R. (2010). Chemistry (10th ed.). New York City, USA: McGraw-Hill Companies, Inc.
Kotz, J., Treichel, P., & Townsend, J. (2015). Chemistry & chemical reactivity (9th ed.). Stamford, CT: Cengage Learning.
Zumdahl, S., Zumdahl, S., & DeCoste, D. (2010). Chemistry (8th ed.). Belmont, California: Brooks Cole.
Next topic: Compounds
Previous topic: Atoms
Return to the main article: The Ultimate Chemistry Reviewer
Download Article in PDF Format
Test Yourself!
1. Practice Questions [PDF Download]
2. Answer Key [PDF Download]
Written by John Bryan Rolloque
in College Entrance Exam, LET, NMAT, Reviewers, UPCAT
John Bryan Rolloque
John Bryan Rolloque graduated cum laude at the University of the Philippines Los Baños in 2018 under the B.S. Agricultural Chemistry program. He taught courses in general chemistry, analytical chemistry, and organic chemistry at UPLB’s Institute of Chemistry, and has been serving as the Region IV coordinator for the Regional and National Chemistry Olympiad. Landing 8th place in the 2019 licensure exam for agriculturists, he is now taking up his master’s degree in plant physiology, also in UPLB.
Copyright Notice
All materials contained on this site are protected by the Republic of the Philippines copyright law and may not be reproduced, distributed, transmitted, displayed, published, or broadcast without the prior written permission of filipiknow.net or in the case of third party materials, the owner of that content. You may not alter or remove any trademark, copyright, or other notice from copies of the content. Be warned that we have already reported and helped terminate several websites and YouTube channels for blatantly stealing our content. If you wish to use filipiknow.net content for commercial purposes, such as for content syndication, etc., please contact us at legal(at)filipiknow(dot)net