Heat and Thermodynamics
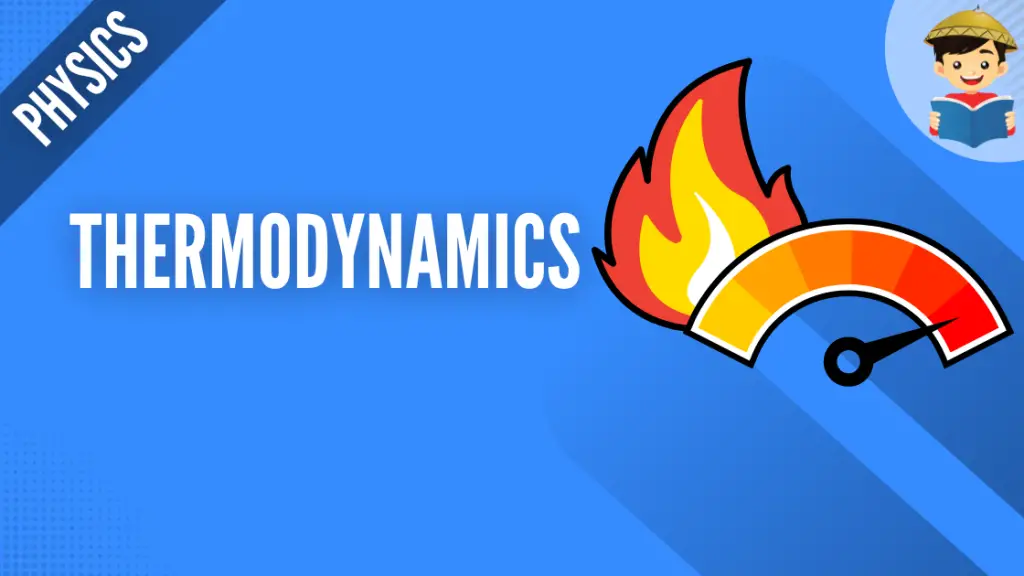
Have you ever wondered how a thermometer works? How do we know when an object is hot or cold? We must understand the concept of heat and thermodynamics to answer these questions.
In this reviewer, we will learn about heat and go through the details of thermodynamics.
Click below to go to the main reviewers:
Table of Contents
- Part I. Heat and Temperature
- Part II. Laws of Thermodynamics
- Download Article in PDF Format
- Test Yourself!
Part I. Heat and Temperature
We all know what heat and temperature are.
When you are asked about the temperature today, you usually describe it as a hot or a cold day. The weather forecasts usually indicate temperature with numbers.
But what does temperature measure? What do these numbers mean?
In physics, heat is an energy in transit, while temperature is a quantity that measures the amount of heat available for work in a system.
It is also related to the average kinetic energy of the molecules in the system. We know that kinetic energy equals ½mv2, which implies that the higher the kinetic energy, the faster the objects move. The same thing can also be applied to the molecules of an object – the faster they move, the greater their kinetic energy, which will result in a higher temperature.
When an object has a high temperature, we usually describe it as hot, and when it has a low temperature, we describe it as cold. This is where the definition of temperature as a degree of hotness or coldness originated from.
Now, how do objects change the temperature?
An object changes its temperature when there is a heat transfer. Heat can be transferred through conduction, convection, and radiation.
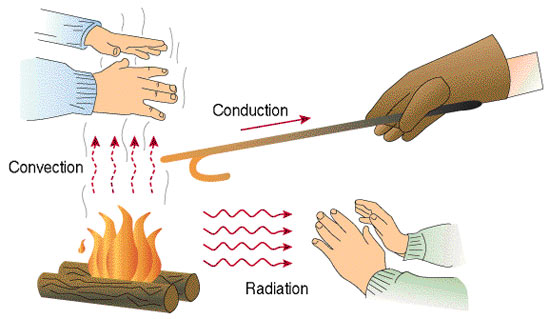
Conduction occurs when the heat is transferred between two objects in contact with one another. An example of conduction is boiling a water kettle on an electric stove. Heat flows from the stove to the kettle, causing the kettle to conduct heat and eventually result in boiling water.
Convection, on the other hand, occurs when the heat is transferred due to the actual motion of the fluid, such as currents.
The mechanism of air-conditioning units is a good example of convection. If you have noticed, most of these are placed at the top of our room. This is because when air is cooled, it becomes denser and sinks. When cold air is sunk, the warmer air at the bottom rises. This process sets up a convection current and will continue unless the temperature changes.
The air-conditioning unit emits cold air, so for this process to work, it must be placed on top to make the room cool. If the unit is placed below, only the bottom air will be cold, and the room would not be cold.
Lastly, radiation transfers heat through electromagnetic waves. This is how your wet clothes get dry when you hang them outside your house on a sunny day. The heat from the sun causes the water in your wet clothes to evaporate and eventually dries your clothes.
The heat will always flow from hot to cold objects since when the particles move, they will transfer some of their kinetic energy to other particles through collision. This is the reason why hot objects feel hot and cold objects feel cold. If you hold a cup of hot tea, the cup will transfer heat into your hands. On the other hand, if you hold a block of ice, the heat will go from your hands to the ice.
This may get confusing, but remember that it will always be from hot to cold and not the other way around. The heat will continue to flow until the system attains the same temperature or thermal equilibrium.
A good example of thermal equilibrium is a thermometer.
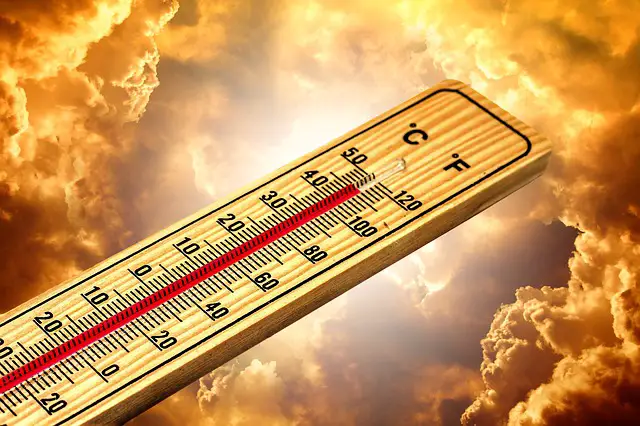
Thermometers are in thermal equilibrium with the objects being measured. When the object comes in contact with the thermometer, the heat coming from the body flows into the thermometer fluid. This allows atoms to gain kinetic energy and columns to expand until it reaches the thermal equilibrium with the body.
All phases of matter expand with higher temperatures. This is known as thermal expansion.
Thermal expansion occurs in solids, liquids, or gases. Solids such as metals usually expand linearly, which results in an increase in length, while liquids and gases result in a volume increase.
Most substances expand when there is an increase in their temperature unless constrained. Aside from the liquid mercury in the thermometer that expands as it measures the temperature of our body, another good example is the opening of a tightly sealed jar of mayonnaise.
When the lid is too tight, we usually run the metal jar lid under hot water to heat it. This causes the metal lid to expand, and when it does, the gap between the lid and the glass increases, resulting in an effortless way of opening a jar.
Internal Energy
We have described temperature as the measurement of average kinetic energy per molecule of a substance. Aside from kinetic energy, molecular potential energy also exists due to the intermolecular and intramolecular bonding of atoms in the objects.
The sum of all types of energies that particles exhibit is called internal energy (U). Internal energy is associated with the motions of the atom and is directly proportional to the temperature. Hence, when the temperature is high, the object has more internal energy, and when the temperature is low, the object has less internal energy.
This concept explains that objects contain internal energy, not heat. It is the transfer of internal energy from high temperatures to low temperatures that counts as heat.
Internal energy can increase due to heat transfer, friction, and deformation.
Part II. Laws of Thermodynamics
Now that we understand how temperature and heat are different from one another and what they mean, we can now proceed with thermodynamics.
Thermodynamics is a branch of physics that studies heat and its transformation into mechanical energy. This field can be summarized by its laws. The first one, called the “Zeroth Law,” tackles the thermal equilibrium, which we discussed in the above section. We will focus on discussing the other laws of thermodynamics.
A. First Law of Thermodynamics
The first law of thermodynamics, also known as the law of energy conservation, relates to heat (Q), work (W), and internal energy (U). This law states that when heat flows to or from a system, the system gains or loses energy equal to the amount of heat transferred. We can state this law in terms of an equation.
ΔU = Q – W
This means that the change in internal energy of a system is equal to the energy transferred to or from the system that counts as heat minus the energy transferred to or from the system as work. No energy has been lost; hence, energy is conserved.
We can also use the concept of this law to describe processes.
When the volume is constant in the system, no work related to pressure and volume can be done on or by the system, hence work will be equal to 0. Therefore, the change in internal energy will equal the heat transfer in and out of the system (ΔU = Q). This process is also known as the isochoric process.
When the temperature is constant, on the other hand, there will be no change in internal energy (ΔU = 0) since they are directly proportional to one another. Thus, the heat transferred to the system will be used to do work. This process is known as the isothermal process.
In the case of no heat transfer (Q = 0), the internal energy changes due to work on or by the surroundings. This process is known as the adiabatic process.
This law can also be used to perform calculations, so we must establish sign conventions.
When heat is absorbed, Q will be positive, while when the heat is lost, Q will be negative. On the other hand, when work is done by the system, such as expansion, work will be positive. When work is done on the system, such as compression, work will be negative. If no work is done or no heat transfer has occurred, the value of the quantity will be 0.
B. Second Law of Thermodynamics
The second law of thermodynamics can be stated in different ways.
Let us start with the easiest one: heat will never flow from cold to hot. The direction of heat flow will always be from hot to cold unless there would be an external source of energy that does work on the system, such as an air-conditioning unit or heat engines.
The second law of thermodynamics came through the study of heat engines by the French engineer Nicolas Leonard Sandi Carnot.
A heat engine is a device that absorbs energy and uses it to do work. When we talk about heat engines, we must know reservoirs. Heat flows into a cold reservoir and out of the hot reservoir. Excess heat that is not used to work would be moved to the cold reservoir.
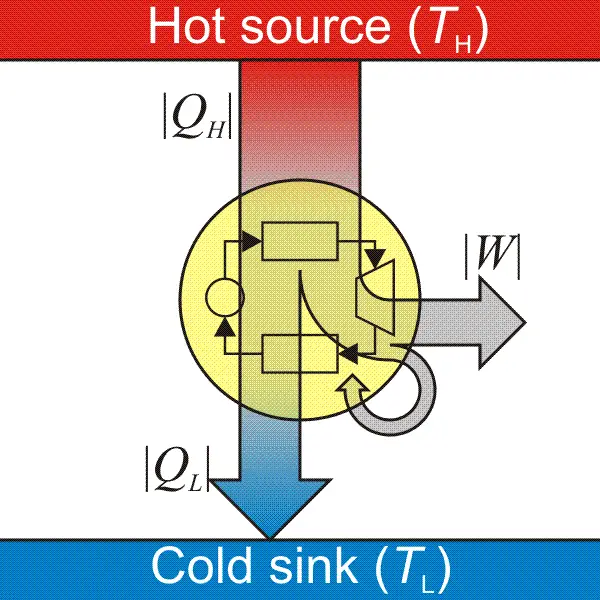
To achieve the engine’s maximum amount of work done in one cycle, heat input will be subtracted from the heat output. To achieve the engine’s maximum efficiency (100%), all of the heat should be transferred to work, but this is not exactly possible since the process must be reversible to achieve 100% efficiency.
When the process is reversible, the system and the surrounding can return to their original states, but since some of the energy has been lost in the surroundings due to friction or sound of the moving engine, the process is irreversible. This became the basis of the second law, which can also be stated as no cyclic process can convert heat into work with 100% efficiency. This relates to our first statement (heat will never flow from cold to hot) since heat cannot flow spontaneously from the cold reservoir to the hot reservoir.
The study of heat engines can eventually lead us to another concept called entropy (S). This is represented by the amount of excess heat in the heat engine. This can also be referred to as the amount of disorderliness in a system that increases as usable energy decreases.
For any reversible process, the change in entropy will be zero. However, since there are no reversible processes, the change in entropy will always be increasing or greater than zero.
We can also relate the first law with the second law of thermodynamics. We know from the first law of thermodynamics that energy will always be conserved, so the quantity of the energy will be constant. However, in the second law of thermodynamics, the energy becomes less useful as time passes, so its quality tends to decrease.
Next topic: Nuclear Physics
Previous topic: Electricity and Magnetism
Return to the main article: The Ultimate Physics Reviewer
Download Article in PDF Format
Test Yourself!
1. Practice Questions [PDF Download]
2. Answer Key [PDF Download]
Written by Mary Joy Montenegro
Mary Joy Montenegro
Mary Joy Montenegro is a licensed professional teacher who obtained her Master’s Degree in Teaching Physics at De La Salle University under the Department of Science and Technology Scholarship. Aside from teaching, she also loves traveling and meeting other people.
Copyright Notice
All materials contained on this site are protected by the Republic of the Philippines copyright law and may not be reproduced, distributed, transmitted, displayed, published, or broadcast without the prior written permission of filipiknow.net or in the case of third party materials, the owner of that content. You may not alter or remove any trademark, copyright, or other notice from copies of the content. Be warned that we have already reported and helped terminate several websites and YouTube channels for blatantly stealing our content. If you wish to use filipiknow.net content for commercial purposes, such as for content syndication, etc., please contact us at legal(at)filipiknow(dot)net